Coastal environment and infrastructure in the Arctic
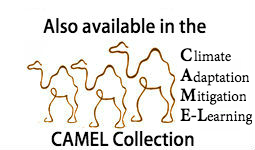
This is Section 6.2.4 of the Arctic Climate Impact Assessment.
Lead Author: Arne Instanes; Contributing Authors: Oleg Anisimov, Lawson Brigham, Douglas Goering, Lev N. Khrustalev, Branko Ladanyi, Jan Otto Larsen; Consulting Authors: Orson Smith, Amy Stevermer, Betsy Weatherhead, Gunter Weller
The Arctic has approximately 200,000 km of coastline, most of which is uninhabited. However, coastal development is critical to the economy and social well-being of nearly all arctic residents (see also Chapters 3 (Coastal environment and infrastructure in the Arctic), 12 (Coastal environment and infrastructure in the Arctic), and 15 (Coastal environment and infrastructure in the Arctic)). Natural-resource development is concentrated along the coast, and the development of resources in these remote areas is constrained because of challenging transportation routes[1].
Arctic coastal dynamics are often affected directly or indirectly by the presence of permafrost. Permafrost coasts are especially vulnerable to erosive processes as ice beneath the seabed and shoreline melts from contact with warmer air and water. Thaw subsidence at the shore allows additional wave energy to reach unconsolidated erodible materials. Low-lying, ice-rich arctic permafrost coasts are the most vulnerable to thaw subsidence and subsequent wave-induced erosion.
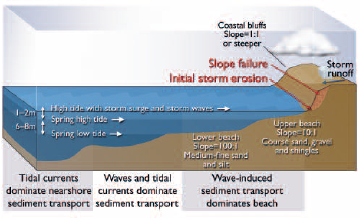
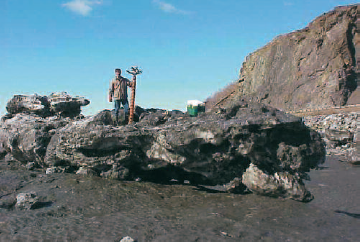
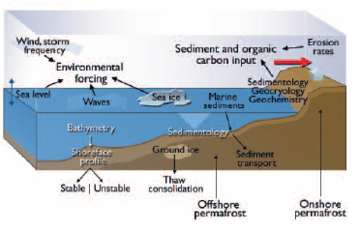
The southern half of Alaska has coastal characteristics dominated by erodible glacial deposits and high tides. Cook Inlet, in south-central Alaska, has a 10 m tidal range at its northern extreme and an eroding shoreline of glacially deposited bluffs, as illustrated in Fig. 16.11[4]. Freezing of brackish water and ice deposition on broad tidal flats create huge blocks of “beach ice” (Fig. 16.12) that, when set afloat by higher tides, can carry coarse sediments for distances of over a hundred kilometers. Of all Cook Inlet sea ice, these sediment-laden ice blocks present the greatest danger to ships in winter. The complex dynamics of bluff erosion and ice-borne sediment transport will become even more difficult to forecast with sea-level rise and a more erratic storm climate.
Contents
Observed changes in the coastal environment (16.2.4.1)
Coastal erosion rates vary considerably across the Arctic. As indicated in Fig. 16.13, erosion rates are dependent on environmental forcing, sedimentology, geocryology, geochemistry, and anthropogenic disturbance of the coastline. In fine-grained icy silty–clayey sediments, average erosion rates are typically 1 to 3 m/yr, while in silty–sandy sediments with high ice content that are directly exposed to waves and storm surges, erosion rates can be as high as 10 to 15 m/yr under extreme weather conditions. Frozen rock and sediments with low ice content may have erosion rates as low as 0.1 m/yr. Anthropogenic disturbance of the coastline can increase erosion rates, but there are also examples from Varandei in the Pechora Sea indicating that shore protection techniques can slow erosion rates. Table 16.8 presents examples of erosion rates along the arctic coast.
Erosion rates have increased along the arctic coast over the past 30 years. Coastal residents are concerned about the observed changes and the future of arctic coastal communities. However, arctic coastal survey data are often inadequate to reliably quantify accelerating shoreline retreat. Baseline surveys using satellite imagery will help the assessment of erosion rates and systematic planning of future responses significantly. Understanding of circumpolar coastal dynamics is also inadequate. Improved understanding of the physical processes involved in arctic coastal erosion will improve techniques for shore protection and other mitigation measures that may be necessary in the future.
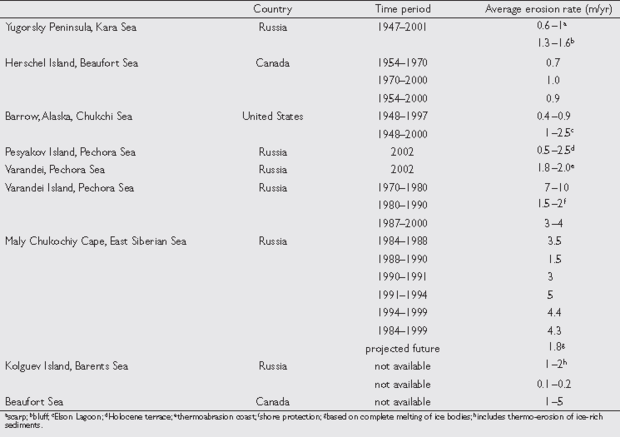
The Arctic Coastal Dynamics project (ACD) is a recent international initiative, sponsored by the International Arctic Sciences Committee and the IPA, to address coastal change in the Arctic[6]. Its objective is to improve the understanding of coastal dynamics as a function of environmental forcing (including climate change), coastal geology and cryology, and changes in landforms (Fig. 16.13). The ACD has proposed:
- to establish the rates and magnitudes of erosion and accumulation along arctic coasts (e.g., Table 16.8);
- to develop a network of long-term monitoring sites including local community-based observation sites; and
- to develop empirical models to assess the sensitivity of arctic coasts to environmental variability and change and human impacts.
Output from studies such as those proposed by the ACD can be used for site-specific evaluation of arctic coastal areas. This type of research and monitoring is essential to document ongoing and future changes and to make policy recommendations.
Projected changes in the coastal environment (16.2.4.2)
Arctic coastal conditions are likely to change as climate changes. Thinner, less extensive sea ice is very likely to improve navigation conditions along most northern shipping routes, such as the Northwest Passage and the Northern Sea Route (see also Section 16.3.7 (Coastal environment and infrastructure in the Arctic)). However, decreasing sea-ice extent and thickness is very likely to affect traditional winter travel and hunting where sea ice has been used for these purposes.
Greater expanses and longer periods of open water are likely to result in wave generation by winds over longer fetches and durations. Wave energy is constrained by wind speed, duration of winds, extent of fetch, and water depth. Wave-induced coastal erosion along arctic shores is likely to increase as climate changes. Sea-level rise and thaw subsidence of permafrost shores are projected to exacerbate problems of increased wave energy at the coast. If more frequent and intense storms accompany climate change, these are also likely to contribute to greater wave energy. Global sea level is rising, although the contribution of various factors to this rise is still being debated (section 6.9.2). The volume of water in the oceans is increasing due to thermodynamic expansion of seawater and melting of ice caps and glaciers. Rising sea level inundates marshes and coastal plains, accelerates beach erosion, exacerbates coastal flooding, and increases the salinity of bays, rivers, and groundwater. Some northern regions, such as the southern coasts of Alaska, have sea-level trends complicated by tectonic rebound of the land resulting from the retreat of continental glaciers at the end of the last glacial period. At Sitka, in south- east Alaska, the net effect is falling sea level. However, arctic coasts have a wide variation of tectonic trends. Low-lying coastal plains in the Arctic are generally not tectonically active, which is another reason why they are vulnerable to the adverse effects of sea-level rise.
Global sea-level rise will possibly allow more wave energy to reach the coast and induce erosion as waves break at the shore.
Reduced sea-ice extent and thickness are very likely to provide opportunities for the export of natural resources and other waterborne commerce over new northern shipping routes. Reduced sea ice in the coastal regions of the Arctic Ocean is very likely to result in longer navigation seasons along the Russian Arctic coast, in the Canadian Arctic, along the eastern and western coasts of Greenland, and around Alaska. Enhanced regional arctic navigation, such between Europe and the Kara Sea, and potential trans-arctic voyages using icebreaking container ships and tankers, is very likely to shorten distances between markets and improve the delivery times for valuable products.
Climate change is also likely to change the use of arctic rivers for transportation routes, water sources, and habitat. Increased precipitation is very likely to result in higher stream flows and more flooding. Conditions for commercial river navigation will possibly improve the transport of minerals and bulk exports to tidewater. Erosion of thawing permafrost banks is very likely to accelerate, threatening the infrastructure of rural arctic river communities. River-ice breakup is very likely to occur earlier and ice jams and flooding risks are likely to be more difficult to project. The projection and prevention of ice-jam flooding warrant further study.
Higher sea levels at the mouths of rivers and estuaries are likely to allow salt to travel further inland, changing riparian habitats. Furthermore, climate change is projected to result in more frequent and intense storms accompanied by stronger winds. These winds are very likely to induce even higher water levels at the coast, accompanied by higher waves. Storms are also likely to result in more intense rainfall at the coast, increasing runoff-related erosion and the mobile sediment in coastal waters.
Coastal communities are sensitive to climate change. Engineering solutions are available for shore protection (flood barriers, dikes, breakwaters, erosion control) but may not be able to reduce erosion rates sufficiently to save specific settlements. Moreover, while these protective measures may address one problem, they may create another by altering the dynamics of erosion and deposition processes.
Chapter 16: Infrastructure: Buildings, Support Systems, and Industrial Facilities
16.1 Introduction (Coastal environment and infrastructure in the Arctic)
16.2. Physical environment and processes related to infrastructure
16.2.1. Observed changes in air temperature
16.2.2. Permafrost (Permafrost and infrastructure in the Arctic)
16.2.3. Natural hazards
16.2.4. Coastal environment
16.2.5. Arctic Ocean (Arctic ocean, climate and infrastructure)
16.3. Infrastructure in the Arctic
16.4. Engineering design for a changing climate
16.5. Gaps in knowledge and research needs (Gaps in knowledge and research needs for infrastructure in the Arctic)
References
Citation
Committee, I. (2012). Coastal environment and infrastructure in the Arctic. Retrieved from http://editors.eol.org/eoearth/wiki/Coastal_environment_and_infrastructure_in_the_Arctic- ↑ Smith O. and Levasseur, 2002, Op. cit.
- ↑ redrawn from Smith, O.P. and G. Levasseur, 2002. Impact of climate change on transportation infrastructures in Alaska. Discussion paper. Department of Transportation Workshop, 1–2 October, 2002, Washington D.C. 11pp.
- ↑ redrawn from Rachold,V., J. Brown and S. Solomon (eds.), 2002. Arctic Coastal Dynamics. Report of an International Workshop, Potsdam, Germany, 26–30 November 2001. Reports on Polar Research 413, 103pp.
- ↑ Ibid.
- ↑ Brown et al., 2003;-- Rachold et al., 2002, Op. cit.;-- Rachold,V., J. Brown, S. Solomon and J.L. Sollid (eds.), 2003. Arctic Coastal Dynamics - Report of the Third International Workshop. Oslo, 2–5 December 2002. Reports on Polar and Marine Research 443, 127pp.
- ↑ Rachold et al., 2002, 2003, Op. cit.