Arctic ocean, climate and infrastructure
This is Section 6.2.5 of the Arctic Climate Impact Assessment.
Lead Author: Arne Instanes; Contributing Authors: Oleg Anisimov, Lawson Brigham, Douglas Goering, Lev N. Khrustalev, Branko Ladanyi, Jan Otto Larsen; Consulting Authors: Orson Smith, Amy Stevermer, Betsy Weatherhead, Gunter Weller
Contents
Observed changes in sea-ice extent (16.2.5.1)
Climatic and environmental changes in the Arctic Basin include changes in air temperature, water temperature and salinity, and the distribution, extent, and thickness of sea ice.There is compelling empirical evidence of consistent environmental changes across the Arctic Ocean, including increases in air temperature, reductions in sea-ice extent, and freshening of the Beaufort Sea mixed layer[1]. Data from ice-floe measurements show a slight air temperature increase with statistically significant warming in May and June between 1961 and 1990. Air temperature anomalies in the Arctic Basin have been strongly positive since 1993. Between 1987 and 1997, the mean annual air temperature increased by 0.9 ºC[2], comparable to temperature changes observed in the terrestrial Arctic.
The area of warm Atlantic waters in the polar basin increased by almost 500,000 km2 over the past three decades[3], and the inflowing freshwater has warmed[4]. Measurements from submarines indicate that surface waters in the Arctic Ocean basin warmed by 0.5 to 1 ºC from the mid-1970s to the mid-1990s, with maximum warming observed in the Kara Sea[5].
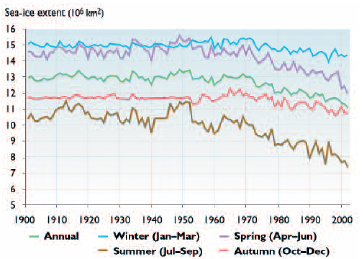
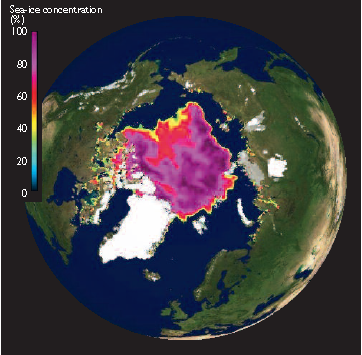
One of the most valuable and graphic records of seaice extent changes in the Northern Hemisphere was produced by Walsh and Chapman[8]. Figure 16.14 shows a 103-year record (1900–2002) of sea-ice extent. A decreasing trend in sea-ice extent, starting in the mid-20th century, is evident for all four seasons. The greatest decreases have occurred in summer and spring: over the past 50 years, summer sea-ice extent has decreased by nearly 3 x 106 km2. Although the decrease in sea-ice extent has been unevenly distributed around the coastal margins of the Arctic Ocean, it has provided greater marine access for ships.
Satellites have recorded increasing areas of open water along the Russian Arctic coast and in the Beaufort Sea. Figure 16.15 is a satellite passive microwave image showing the extent of arctic sea ice on 22 September 2002 – the date of the minimum observed extent in the 103-year record. This image illustrates the large areas of open water surrounding the Arctic Basin at the summer minimum extent of sea ice. Of significance for the Northern Sea Route, the only pack ice reaching the Russian Arctic coast is along the northern tip of Severnaya Zemlya; the sea ice in this image has also retreated record distances in the Beaufort, Chukchi, and East Siberian Seas.
Sea-ice conditions in the Canadian Arctic are very complex. Observations of minimum sea-ice extent in the eastern and western regions of the Canadian Arctic between 1969 and 2003 (Fig. 16.16) illustrate the extraordinary interannual variability of the ice conditions. Although the trends in sea-ice extent are negative in both regions over the period shown, the year-to- year variability is extreme and sometimes differs between the two regions. For example, one of the largest observed minimum extents in the western region (for the period shown) occurred in 1991, while in the eastern region the minimum sea-ice extent that year was relatively low. While these observations indicate a recent overall decrease in the extent of sea ice in the Northwest Passage, the interannual and spatial variability is not conducive to planning a reliable marine transportation system.
Projected changes in sea-ice extent (16.2.5.2)
Figure 16.17 shows the median of the sea-ice extents projected by the five ACIA-designated climate models for the three ACIA time slices. However, an important limitation of the ACIA-designated models is that they cannot resolve the complex geography of the Canadian Arctic and thus cannot provide adequate sea-ice projections for this region. In summer, the models project a substantial retreat of sea ice throughout the entire Arctic Ocean for each ACIA time slice, except for parts of the Canadian Archipelago and along the northern coast of Greenland. By mid-century (September 2041–2060), most of the alternative routes in the Northwest Passage and Northern Sea Routeare projected to be nearly ice-free; three of the five models project open water conditions across the entire lengths of both. By the end of the 21st century, vast areas of the Arctic Ocean are projected to be ice-free in summer, increasing the possibility of shipping across the Arctic Ocean.
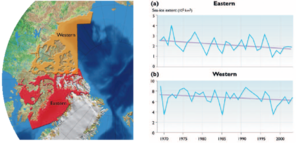
Although there is some projected winter retreat of the sea-ice edge, particularly in the Bering and Barents Seas where ship access is likely to improve throughout the 21st century, most of the Arctic Ocean is projected to remain ice-covered in winter. However, sea-ice cover is likely to be on average thinner, and the area of multi-year sea ice in the central Arctic Ocean is likely to decrease through 2080.Winter navigation (throughout the year to Dudinka since 1978) along the western end of the Northern Sea Route, from the Barents Seato the mouths of the Ob and Yenisey Rivers, will possibly encounter less first-year sea ice. Full transit of the Northern Sea Route through Vilkitskii Strait to the Bering Sea is very likely to remain challenging and require icebreaker escort. The only improvement in winter sea-ice conditions for the Laptev, East Siberian, and western Chukchi Seas is a possible reduction in the area and frequency of multi-year floes along the navigable coastal routes.
The significant interannual sea-ice variability in the arctic seas is also important to navigation. The projected increase in ice-free areas and reduced sea-ice concentrations will possibly lead to a more dynamic sea ice cover under the influence of local and regional wind fields.These more variable environmental conditions, combined with an increase in the number of ships making passages in the Arctic Ocean, will possibly lead to a higher demand for sea-ice information, long range ice forecasting, and increased icebreaker support. While direct (across the North Pole) trans-arctic voyages are unlikely by mid-century, voyages may be possible north of the arctic island groups (such as those along the Russian Arctic coast) and away from shallow continental shelf areas that restrict navigation.
There is likely to be an increased requirement for realtime satellite imagery of sea-ice conditions. Increased ship access to the Arctic Ocean is very likely to require more resources to facilitate marine traffic and to support maritime safety and protection of the arctic marine environment.
The four ACIA regions are not conveniently drawn for assessment of future arctic marine transport routes. Therefore, as a case study, a new “sector” was defined between 60º and 90º N, and between 40º E and 170º W, which includes the coastal region from the eastern Barents Sea to Bering Strait. This sector also encompasses Russia’s Northern Sea Route, defined by regulation as the routes or waterways between Kara Gate (at the southern end of Novaya Zemlya) and Bering Strait.
As shown in Fig. 16.17, the median of the ACIAdesignated model projections suggests that, in all the time slices, most coastal waters of the Eurasian Arctic (along the Northern Sea Route) will be relatively icefree in September. Figure 16.18 illustrates the September sea-ice extents projected by each of the five models for the Eurasian Arctic sector used in this analysis. During September, the month of minimum sea-ice extent in the Arctic Ocean, the models consistently project sea ice in the vicinity of Severnaya Zemlya during each of the ACIA time slices. For ships to travel north of Severnaya Zemlya, highly capable, more expensive icebreaking ships would be necessary. However, it is more likely that ships would sail through the deep waters of Vilkitskii Strait to the south – between the Kara and Laptev Seas north of the Taymir Peninsula – where more open water is very likely to be found. Four of the five models also project open water to the east and north of the New Siberian Islands in September. Ships sailing along the Northern Sea Route are likely to take advantage of these ice-free conditions to avoid the shallow, narrow passages along the Eurasian coast. In addition, if there is a continued reduction in the proportion of winter multi-year sea ice in the Central Arctic Ocean[10], it is very likely that first-year sea ice will dominate the entire maritime region of the Northern Sea Route throughout the year, with a decreasing frequency of intrusions of multi-year sea ice into the coastal seas. Such changes in sea-ice conditions would have key implications for ship construction (e.g., potentially lower construction costs) and route selection along the Northern Sea Route in summer and winter.
Chapter 16: Infrastructure: Buildings, Support Systems, and Industrial Facilities
16.1 Introduction (Arctic ocean, climate and infrastructure)
16.2. Physical environment and processes related to infrastructure
16.2.1. Observed changes in air temperature
16.2.2. Permafrost (Permafrost and infrastructure in the Arctic)
16.2.3. Natural hazards
16.2.4. Coastal environment
16.2.5. Arctic Ocean
16.3. Infrastructure in the Arctic
16.4. Engineering design for a changing climate
16.5. Gaps in knowledge and research needs (Gaps in knowledge and research needs for infrastructure in the Arctic)
References
Citation
Committee, I. (2012). Arctic ocean, climate and infrastructure. Retrieved from http://editors.eol.org/eoearth/wiki/Arctic_ocean,_climate_and_infrastructure- ↑ Maslanik, J.A., M.C. Serreze and R.G. Barry, 1996. Recent decreases in Arctic summer ice cover and linkages to atmospheric circulation anomalies. Geophysical Research Letters, 23:1677–1680.;--McPhee, M.G.,T.P. Stanton, J.H. Morison and D.G. Martinson, 1998. Freshening of the upper ocean in the Central Arctic: Is perennial sea ice disappearing? Geophysical Research Letters, 25:1729–1732.
- ↑ Aleksandrov,Ye.I. and V.V. Maistrova, 1998. Comparison of the air temperature measurements for the polar regions. The Antarctica, 34:60–72.
- ↑ Kotlyakov,V.M., 1997. Quickly warming Arctic basin. The Earth and the World, 4:107.
- ↑ Carmack, E.C., 2000. Review of the Arctic Ocean’s freshwater budget: sources, storage and export. In: E.L. Lewis, E.P. Jones, P. Lemke,T.D. Prowse and P.Wadhams (eds.). The Freshwater Budget of the Arctic Ocean. Kluwer Academic Publishers.-- Carmack, E.C., R.W. Macdonald, F.A. Perkin, F.A. McLaughlin and R.J. Pearson, 1995. Evidence for warming of Atlantic water in the southern Canadian basin of the Arctic Ocean: Results from the Larsen-93 Exhibition. Geophysical Research Letters, 22:1061–1064.
- ↑ Alekseev, G.V., Z.V. Bulatov,V.F. Zakharov and V.P. Ivanov, 1997. Influx of very warm water in the Arctic basin. Doklady Akademii Nauk SSSR, 356:401–403.
- ↑ Walsh, J.E. and W.L. Chapman, 2001.Twentieth-century sea-ice variations from observational data. Annals of Glaciology, 33:444–448., updated
- ↑ figure provided by William Chapman, University of Illinois, 2003
- ↑ Walsh and Chapman, 2001, Op. cit.
- ↑ redrawn from figure provided by the Canadian Ice Service, 2004
- ↑ Johannessen, O., E. Shalina and M. Miles, 1999. Satellite evidence for an Arctic sea ice cover in transformation. Science, 286:1937–1939.