Snow cover in the Arctic
This is Section 6.4 of the Arctic Climate Impact Assessment.
Lead Author: John E.Walsh; Contributing Authors: Oleg Anisimov, Jon Ove M. Hagen,Thor Jakobsson, Johannes Oerlemans,Terry D. Prowse,Vladimir Romanovsky, Nina Savelieva,Mark Serreze, Alex Shiklomanov, Igor Shiklomanov, Steven Solomon; Consulting Authors: Anthony Arendt, David Atkinson, Michael N. Demuth, Julian Dowdeswell, Mark Dyurgerov, Andrey Glazovsky, Roy M. Koerner, Mark Meier, Niels Reeh, Oddur Sigur0sson, Konrad Steffen, Martin Truffer
Contents
Background (6.4.1)
Terrestrial snow cover is the most rapidly varying cryospheric variable on the surface of the earth. An individual frontal cyclone (Tropical weather and hurricanes) can change the area of snow-covered land (or sea ice) by 0.1 to 1.0 million square kilometers (km2) in a matter of days. Snow cover also displays large spatial variability in response to wind, and to topographic and vegetative variations.Yet it is the spatially integrated accumulation of snow over one to two seasons that has important hydrological implications for arctic terrestrial regions and the polar oceans, and hence for terrestrial and marine ecosystems. Snow also represents the fundamentally important accumulation component of ice sheets and glaciers (Section 6.5 (Snow cover in the Arctic)). Finally, snow cover influences the ground thermal regime and therefore the permafrost changes (Section 6.6 (Snow cover in the Arctic)) that have additional hydrological implications (Section 6.8 (Snow cover in the Arctic)).
Before the availability of satellite imagery in the 1960s, snow cover was determined from occasional aerial photographs and from point measurements, often made at weather stations spaced irregularly over the land surface. In cold and [[wind]y] environments such as the Arctic, point measurements are inaccurate because snow gauges are inefficient and drifting snow contaminates the measurements[1]. In addition, even accurate point measurements may not be representative of large-area or regional snow-cover conditions. The inaccuracy of the point measurements makes them inadequate for mapping the detailed spatial structure of snow coverage and depth, especially in regions of significant topography. Because snow cover is easily identified in visible and near-infrared wavelength bands, owing to its high reflectance, satellites have proven valuable in monitoring variations in snow cover at various scales over the past three to four decades. Unfortunately, most sensors cannot measure snow depth or water equivalent[2].
The present distribution of snow cover in the Northern Hemisphere, excluding permanently glaciated areas such as Greenland, varies from <1 million km2 in late August to 40 to 50 million km2 in February[3]. The large range in the February values indicates the interannual variability. Figure 6.11 shows the frequency of snow cover on the land areas of the Northern Hemisphere from 1966 to 2000 during winter (December), early and late spring transition months (February and May), and an autumn transition month (October). It is apparent that snow is a quasipermanent feature of the arctic terrestrial landscape during winter. The variability inherent in subarctic land areas during the spring (Fig. 6.11b), when insolation is relatively strong, implies that the timing of the snowmelt, which reduces the surface albedo by 20 to 60%, can strongly affect surface absorption of solar radiation. Models have demonstrated the importance of snow cover for the surface energy budget, soil temperature, and the permafrost active layer[4]. Snow cover is also highly variable in October (Fig. 6.11c), when insolation and hence the potential for snow to affect the surface absorption of solar radiation is weaker. Snow is rarely present over the subarctic land areas in July and August.
The hydrologically important characteristic of snow cover is its water equivalent, since this moisture is eventually released to the atmosphere by sublimation or evaporation, or to the polar oceans by runoff. Some of the snow water is siphoned off for human use prior to its eventual release to the atmosphere or ocean. Estimated fields of snow water equivalent (or snow depth) can be derived from satellite passive microwave measurements[5]. Although the spatial coverage of these measurements is complete and their broad spatial patterns are correct, there are large uncertainties and errors for areas in which vegetative masking (vegetation obscuring the underlying snow, making the ground appear darker) is significant (e.g., the boreal forests of the subarctic). Even allowing for the uncertainties, the derived snow water equivalents represent large water supplies that are released to other parts of the climate system during spring melt.
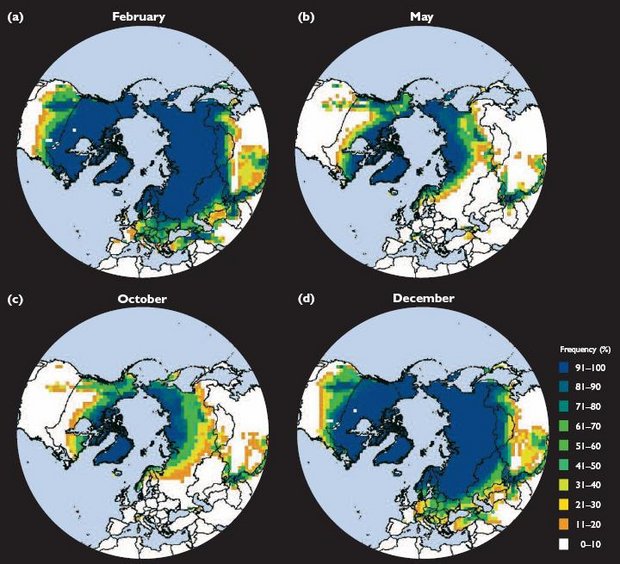
Station-derived climatologies of snow depth represent alternatives to the satellite-derived estimates and their associated uncertainties. Such climatologies have been compiled for Canada[7] and for Russia[8]. However, these compilations are subject to elevation and location related biases in the station networks. Section 6.4.2 (Snow cover in the Arctic) summarizes broad-scale variations in these trends. Radionov et al.[9] compiled statistics of snow depth and duration over the central Arctic Ocean using measurements obtained at the Russian drifting ice stations, primarily between the 1950s and 1990.
Over glaciated regions, where snow stakes and altimetry are major sources of information, the problem of spatial integration of snow measurements is quite different from that over regions of seasonal snow cover. [[Section 6.5 (Snow cover in the Arctic)]2] discusses mass-balance measurements for glaciers and ice caps on a regional basis.
Albedo is highly relevant to the role of snow in the surface thermal regime. The albedo of snow-covered land areas is highly variable, depending on snow depth, snow age, and the masking characteristics of vegetation. There are few systematic compilations of surface albedo over snow cover, so the climatology and variability of surface albedo are not well documented at the circumpolar scale. Winther[10] and others have studied albedo variations on a regional basis.
Snow is a key variable in the rates of soil warming and permafrost thawing. Because snow effectively insulates the upper soil layers during winter, increases in snow depth generally result in higher soil temperatures during the cold season, while an absence of snow results in more rapid and greater cooling of the soil. If snowfall changes substantially as climate changes, warming and thawing or cooling and freezing may significantly affect the upper soil layers. Permafrost models ([[Section 6.6 (Snow cover in the Arctic)]2]) require information on snow cover in addition to air temperature if they are to provide valid simulations of variations in the temperature and water phase in the upper soil layers. In general, climate models treat the subsurface effects of snow rather crudely, particularly with regard to the freeze-thaw cycle of soils over seasonal to centennial timescales.
Recent and Ongoing Changes (6.4.2)
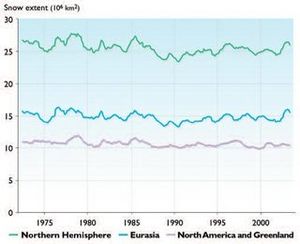
Over a few months, snow cover in the Northern Hemisphere (excluding sea ice, Greenland, and glaciers) varies between the 0 to 5 million km2 typical of summer and the more than 40 million km2 typical of winter. The rapidity of the expansion and retreat (melt) is comparable in the autumn and spring, and indicates the short timescales for variations in snow cover relative to other cryospheric variables. Interannual variations are also rapid. Figure 6.12, for example, shows the 12-month running means of snow extent between 1972 and 2003, the period of homogeneous visible satellite data.While these fluctuations complicate the detection and interpretation of trends, least-squares fits to the time series in Fig. 6.12 indicate that the areal coverage of snow has decreased over the past few decades. The decrease for the Northern Hemisphere is nearly 10% over the period 1972 to 2003. Both North America and Eurasia show decreases, although the decrease is greatest for Eurasia. However, the decrease is highly seasonal, varying from no significant change in autumn and winter to decreases greater than 10% in spring and summer (Fig. 6.13). The large areal decrease in spring is correlated with the large spring warming over the northern land areas (Section 2.6.2 (Snow cover in the Arctic)). The summer trend, to which the arctic land areas are probably making key contributions, has received little attention in the literature.
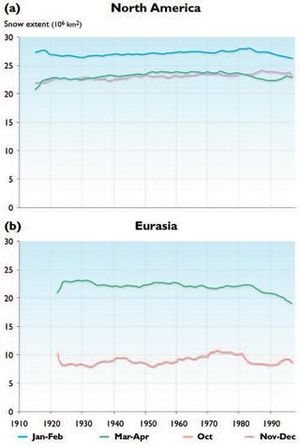
For the period before satellite data were available, variations and trends in snow cover have been assessed largely on a regional basis. North American snow cover shows a general decrease in spring (March–April) extent since the 1950s (Fig. 6.13a), although there are indications that spring snow extent increased during the earlier part of the 20th century. The spring decrease is also apparent in the Eurasian data (Fig. 6.13b). The total extent of Northern Hemisphere snow during spring and summer was lower in the 1990s than at any time in the past 100 years[13]. However, the longer records shown in Fig. 6.13 do not indicate a systematic decrease in snow cover during autumn or early winter for either landmass.
Recent variations in snow depth are more difficult to assess because of measurement and remote-sensing difficulties in vegetated areas. For the pre-satellite era, the sparseness of the synoptic station network precluded systematic mapping of snow depth in many high-latitude areas. Nevertheless, there have been compilations and analyses of snow-depth data for particular regions. Snow depth appears to have decreased over much of Canada since 1946, especially during spring[14]. Winter snow depths have decreased over European Russia since 1900[15], but have generally increased elsewhere over Russia during the past few decades[16], in agreement with the increase in [precipitation]] noted in Section 6.2.2 (Snow cover in the Arctic). Ye H.[17] reports a small (several day) increase in the snow-season length, due primarily to later snowmelt, over north-central and northwest Asia Fig. 6.12. Twelve-month running mean of snow extent in the Northern Hemisphere from 1972 to 2003, showing the entire hemisphere, North America and Greenland, and Eurasia[18] for the period 1937 to 1994. However, this study excludes the years since 1994, during which northern Asia has been relatively warm in winter and spring.
Over the central Arctic Ocean, measurements at Russian North Pole ice stations from the mid-1950s to 1990 suggest a decrease in snow depth, although considerable variability is superimposed on this decrease[19]. Measurements of total precipitation at the same sites show little indication of any systematic trend[20].
Thus, there are consistent indications of spring warming of arctic terrestrial regions (Section 2.6.2.1 (Snow cover in the Arctic)) and earlier disappearance of snow cover. Associated changes are the earlier breakup of ice-covered lakes and rivers (Section 6.7 (Snow cover in the Arctic)) and a seasonal advance (from early spring to late winter) in the timing of the primary pulse of river discharge in the Arctic (Section 6.8 (Snow cover in the Arctic) 2).
Projected Changes (6.4.3)
Simulations of 21st-century climate from the five ACIA designated climate models (Section 4.4 (Snow cover in the Arctic)) were examined for projected changes in snow cover. These changes are complicated by the conflicting effects of higher [[temperature]s], which will result in a poleward retreat of the snow margin, but which are also likely to contribute to an acceleration of the hydrological cycle and –-in those regions that remain consistently below freezing-– an increase in snowfall and possibly snow depth (water equivalent).
Table 6.4 summarizes the changes in snow cover projected by the five ACIA designated models, including snow cover simulated for the baseline climate (1981–2000) and the changes averaged over each of the three ACIA time slices: 2011–2030, 2041–2060, and 2071–2090. It is apparent that the projected changes in mean annual snow cover are not large, even by 2071–2090 when the changes range from -9 to -18%.
Table 6.4. Northern Hemisphere mean annual snow cover simulated by the five ACIA-designated models for baseline climate (1981–2000) and percentage change from the baseline projected for each of the ACIA time slices. | ||||
Snow cover (106 km2) |
Percentage change from baseline(1981–2000) | |||
1981–2000 |
2011–2030 |
2041–2060 |
2071–2090 | |
CGCM2 |
27.8 |
-7 |
-13 |
-17 |
CSM_1.4 |
23.8 |
-3 |
-5 |
-9 |
ECHAM4/OPYC3 |
18.6 |
-6 |
-13 |
-18 |
GFDL-R30_c |
31.9 |
-4 |
-7 |
-10 |
HadCM3 |
23.0 |
-4 |
-8 |
-10 |
Observed |
23.2 |
However, there is a notable seasonality to the changes in snow cover. Table 6.5 summarizes the changes projected to occur between the baseline (1981–2000) and 2071–2090 by season. While the percentage changes are greatest in summer (when areal coverage is very small in the present climate), the actual decrease in snow-covered area is greatest in spring (April and May).
Table 6.5. Seasonal change in snow extent (106 km2)between 1981–2000 and 2071–2090 projected by the five ACIA-designated models. | ||||||
---|---|---|---|---|---|---|
Winter (Dec–Feb) | Spring (Mar–May) | Summer (Jun–Aug) | Autumn (Sep–Nov) | |||
CGCM2 | -5.8 | -6.8 | -3.2 | -3.2 | ||
CSM_1.4 | -2.8 | -3.2 | -0.4 | -2.2 | ||
ECHAM4/OPYC3 | -5.5 | -6.2 | -0.6 | -3.4 | ||
GFDL-R30_c | -2.3 | -5.1 | -0.6 | -4.6 | ||
HadCM3 | -2.8 | -3.1 | -0.5 | -3.0 | ||
Five-model mean | -3.8 | -4.9 | -1.1 | -3.3 |
A more detailed evaluation (Table 6.6) shows that the months with the largest projected reductions in snow extent are April and November, followed by May, March, and December. The changes most relevant to arctic hydrology are those that occur in spring, when a reduction in snow cover implies an earlier pulse of river discharge to the Arctic Ocean and coastal seas. That the greatest projected changes occur during the spring, late autumn, and early winter indicates a shortened snow season in the model simulations for the late 21st century. Figure 6.14 illustrates the distribution of snow cover during the 2071–2090 time slice for March, May, October, and December. A comparison with the present day frequency distributions for May, October, and December (Fig. 6.11) shows that the snow retreat is modest but visually noticeable, especially in May.
Table 6.6. Five-model monthly means of the projected change in snow extent (106km2)between 1981–2000 and 2071–2090. | ||||||||||||
---|---|---|---|---|---|---|---|---|---|---|---|---|
Jan | Feb | Mar | Apr | May | Jun | Jul | Aug | Sep | Oct | Nov | Dec | |
Change in extent | -3.7 | -3.8 | -4.2 | -5.4 | -5.0 | -2.1 | -0.9 | -0.3 | -1.2 | -3.6 | -5.1 | -4.0 |
Impacts of Projected Changes (6.4.4)
On Other Parts of Physical System
The primary effects of a reduction in snow cover will be on the surface energy budget (hence soil temperature and permafrost) and on the surface moisture budget (runoff, evaporation). However, the effects of changes in snow cover will vary seasonally. During winter, snow insulates the ground, so a reduction in snow cover or depth will lead to cooling of the underlying ground. During spring, a decrease in snow cover will lower the surface albedo, leading to enhanced absorption of solar radiation and warming of the ground. For sea ice, decreased snow cover will accelerate ice melt in the spring while increased snow cover will retard ice melt.
Changes in snow cover also have the potential to influence significantly the distribution of vegetation[21], which can then influence the atmosphere through changes in vegetative masking, surface albedo, and the surface energy budget. Snow cover also affects the exchange of Greenhouse Gases (GHGs) between the land surface and the atmosphere, as documented in the Land Arctic Physical Processes experiment[22].
On Ecosystems
The growth season of high-latitude vegetation, and hence primary production and carbon dioxide (CO2) uptake, depends strongly on the timing of snow disappearance, which in turn depends on antecedent snow accumulation. Snow insulates underlying vegetation and other biota (e.g., mammals, insects). The runoff pulse produces large biogeochemical fluxes from terrestrial to marine ecosystems, and changes in the spring snowmelt will affect both the timing and intensity of this pulse. An important characteristic of snow cover is its structure, especially the presence of ice layers that can result from thaw–freeze cycles or from freezing rain events. The presence of ice layers can severely hamper winter grazing by wildlife (Section 12.2.4 (Snow cover in the Arctic)). Unfortunately, neither the observational database nor the model output can provide useful information on the presence of ice layers in snow, although it is reasonable to assume that a warming climate will increase the frequency of winter freeze–thaw cycles and freezing rain events in arctic terrestrial regions.
On People
Changes in the amount of snow will have impacts on transportation (e.g., feasibility, safety, costs); recreation activities and the businesses dependent on them (e.g., ski resorts, snow machines); snow loading on structures and removal costs; avalanche hazards in areas with steep topography; and water supplies for various population sectors. Because the costs of clearing snow from roads are significant in many mid- and high-latitude communities, economic consequences of changes in the amount of snow are very likely. In addition, changes in the length of the snow-free season would affect agricultural, industrial, and commercial activities as well as transportation in many high-latitude communities. Changes in the amount of snow and the length of the snow season will also directly affect the subsistence activities of indigenous communities.
Critical Research Needs (6.4.5)
Global climate model simulations of snow extent have shown some improvement over the past decade[23]. However, a climatology of the spatial distribution of snow water equivalent in each month is a critical need for model validation and hydrological simulations. This is especially urgent for high latitudes, where there are few in situ measurements of snow water equivalent to complement the estimates derived from passive microwave measurements. Information on snow albedo over northern terrestrial regions, especially for vegetated areas and for the late winter and spring seasons when the timing of snowmelt is hydrologically critical, is an additional requirement. Global daily snow-albedo products derived from the Moderate-Resolution Imaging Spectroradiometer are now available[24]; such products should be used for quantitative assessments of large-scale albedo variations and for climate model validation.
The ability of models to simulate the snowmelt process also needs further investigation within the context of arctic hydrology. This should result in evaluations of feedbacks between the timing of snowmelt and broader changes in terrestrial ecosystems.
A potentially important but often overlooked process is the sublimation of snow, especially when enhanced by blowing snow. Sublimation can be a key part of the hydrological cycle locally and regionally[25], yet climate models do not include the enhancement of sublimation by blowing snow, and some models do not include even the direct sublimation of snow from the surface.
Chapter 6: Cryosphere and Hydrology
6.1. Introduction (Snow cover in the Arctic)
6.2. Precipitation and evapotranspiration
6.3. Sea ice (Sea ice in the Arctic)
6.4. Snow cover
6.5. Glaciers and ice sheets
6.6. Permafrost (Permafrost in the Arctic)
6.7. River and lake ice
6.8. Freshwater discharge
6.9. Sea-level rise and coastal stability
See Also
References
Citation
- ↑ Goodison, B.E. and D.Q. Yang, 1996. In-situ measurements of solid precipitation in high latitudes: the need for correction. Proceedings of the workshop on the ACSYS Solid Precipitation Climatology Project, WCRP-93, WMO/TD No.739 3–17pp.
- ↑ Dankers, R. and S.M. De Jong, 2004. Monitoring snow-cover dynamics in northern Fennoscandia with SPOT Vegetation Images. International Journal of Remote Sensing, 25(15):2933–2949.
- ↑ Ramsay, B.H., 1998. The interactive multisensor snow and ice mapping system. Hydrological Processes, 12:1537–1546.–Robinson, D. A., 1993. Hemispheric snow cover from satellites. Annals of Glaciology, 17:367–371.
- ↑ Ling, F. and T. Zhang, 2003. Impact of the timing and duration of seasonal snow cover on the active layer and permafrost in the Alaskan Arctic. Permafrost and Periglacial Processes, 14:141–150.–Sokratov, S. A. and R.G. Barry, 2002. Intraseasonal variations in the thermoinsulation effect of snow cover on soil temperatures and energy balance. Journal of Geophysical Research, 107(D9–10),ACL 13 1–7.
- ↑ Armstrong, R. and M. Brodzik, 2001. Validation of passive microwave snow algorithms. In: M. Owe, K. Brubaker, J. Ritchie and A. Rango (eds.). Remote Sensing and Hydrology 2000. International Association of Hydrological Sciences, Publ. No. 267, pp. 87–92.–Chang, A. T.C., J.L. Foster and D.K. Hall, 1987. Nimbus-7 derived global snow cover parameters. Annals of Glaciology, 9:39–44.–Goodison, B.E. and A.E. Walker, 1995. Canadian development and use of snow cover information from passive microwave satellite data. In: B. T. Choudhury,Y.H. Kerr, E.G. Njoku and P. Pampaloni (eds.). Passive Microwave Remote Sensing of Land-Atmosphere Interactions, pp. 245–261. VSP BV Zeitt, Netherlands.
- ↑ Robinson, D., 2003. Snow Data Resource Center, Rutgers University Climate Laboratory, Piscataway, New Jersey.
- ↑ Brown, R.D. and R.O. Braaten, 1998. Spatial and temporal variability of Canadian monthly snow depths. Atmosphere-Ocean, 36:37–45.
- ↑ Ye, H., H. Cho and P.E. Gustafson, 1998. The changes in Russian winter snow accumulation during 1936–1983 and its spatial patterns. Journal of Climate, 11:856–863.
- ↑ Radionov, V.F., N.N. Bryazgin and E.I. Alexandrov, 1997. The Snow Cover of the Arctic Basin. Tech. Rep. APL-UW TR 9701. Applied Physics Laboratory, University of Washington, Seattle, 95pp.
- ↑ Winther, J.-G., 1993. Short- and long-term variability of snow albedo. Nordic Hydrology, 24:199–212.
- ↑ Robinson, D., 2003. Snow Data Resource Center, Rutgers University Climate Laboratory, Piscataway, New Jersey.
- ↑ Brown, R.D., 2000. Northern Hemisphere snow cover variability and change, 1915–1997. Journal of Climate, 13:2339–2355.
- ↑ IPCC, 2001. Climate Change 2001: The Scientific Basis. Contribution of Working Group I to the Third Assessment Report of the Intergovernmental Panel on Climate Change. J. T. Houghton, Y. Ding, D.J. Griggs, M. Noguer, P.J. van der Linden, X. Dai, K. Maskell and C. A. Johnson (eds.). Cambridge University Press, 881pp.
- ↑ Brown, R.D. and R.O. Braaten, 1998. Spatial and temporal variability of Canadian monthly snow depths. Atmosphere-Ocean, 36:37–45.
- ↑ Meshcherskaya, A. V., I.G. Belyankina and M.P. Golod, 1995. Monitoring tolshching cnozhnogo pokprova v osnovioi zerno proizvodyaschei zone Byvshego SSSR za period instrumental?nykh nablyugenii, Izvestiya Akad. Nauk SSR, Sser. Geograf., 101–110.
- ↑ Fallot, J.-M., R.G. Barry and D. Hoogstrate, 1997. Variations of mean cold season temperature, precipitation and snow depths during the last 100 years in the Former Soviet Union (FSU). Hydrological Sciences Journal, 42:301–327.
- ↑ Ye, H., 2001. Increases in snow season length due to earlier first snow and later last snow dates over north central and northwest Asia during 1937–94. Geophysical Research Letters, 28:551–554.
- ↑ Robinson, D., 2003. Snow Data Resource Center, Rutgers University Climate Laboratory, Piscataway, New Jersey.
- ↑ Radionov, V.F., N.N. Bryazgin and E.I. Alexandrov, 1997. The Snow Cover of the Arctic Basin. Tech. Rep. APL-UW TR 9701. Applied Physics Laboratory, University of Washington, Seattle, 95pp.
- ↑ Radionov, V.F., N.N. Bryazgin and E.I. Alexandrov, 1997. The Snow Cover of the Arctic Basin. Tech. Rep. APL-UW TR 9701. Applied Physics Laboratory, University of Washington, Seattle, 95pp.
- ↑ Bruland, O. and E. Cooper, 2001. Snow distribution and vegetation. In: P. Kuhry (ed.). Proceedings of Arctic Feedbacks to Global Change. Rovaniemen Paintuskeskus Oy, Rovaniemi, Finland, pp. 110.
- ↑ Anon, 1999. Final Report. Land Arctic Physical Process (LAPP). Contract No. ENV4-CT95-0093. 1 April 1996 to 31 March 1999. 134pp.
- ↑ Frei, A., J. A. Miller and D. A. Robinson, 2003. Improved simulations of snow extent in the second phase of the Atmospheric Model Intercomparison Project (AMIP-2). Journal of Geophysical Research, 108(D12):4369, doi:10.1029/2002JD003030.
- ↑ Hall, D.K., G. A. Riggs,V. V. Salomonson, N.E. DiGirolamo and K.J. Bayr, 2002. MODIS snow-cover products. Remote Sensing of Environment, 83:181–194.
- ↑ Pomeroy, J. W. and L. Li, 2000. Prairie and Arctic areal snow cover mass balance using a blowing snow model. Journal of Geophysical Research, 105(D21):26610–26634.