Past variability in Arctic marine systems
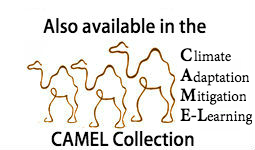
This is Section 9.3.3 of the Arctic Climate Impact Assessment
Lead Author: Harald Loeng; Contributing Authors: Keith Brander, Eddy Carmack, Stanislav Denisenko, Ken Drinkwater, Bogi Hansen, Kit Kovacs, Pat Livingston, Fiona McLaughlin, Egil Sakshaug; Consulting Authors: Richard Bellerby, Howard Browman,Tore Furevik, Jacqueline M. Grebmeier, Eystein Jansen, Steingrimur Jónsson, Lis Lindal Jørgensen, Svend-Aage Malmberg, Svein Østerhus, Geir Ottersen, Koji Shimada
Previous data collections combined with present-day models shows that climate variability is very likely to have influenced population parameters of marine organisms, especially fish (section 9.3.2.3 (Past variability in Arctic marine systems)). Water temperature undoubtedly affects species composition in different areas, as well as the recruitment, growth, distribution, and migration of different fish species. However, most of the relationships between water temperature and population variables are qualitative and few of those discussed here can be quantified.
Contents
Plankton (9.3.3.1)
There are few long time series for phytoplankton in the Arctic. Exceptions include (1) datasets covering 20 years or more for Icelandic waters[1] and Norway (Oslofjord, Trondheimsfjord)[2]; (2) a program undertaken during the 1990s to monitor harmful algae along the Norwegian coast[3]; and (3) zooplankton data provided by the Continuous Plankton Recorder, which has been used in much of the North Atlantic between 50° and 65° N for over fifty years[4]. This has generated one of the most detailed records of seasonal, interannual, and decadal variability in zooplankton to date. Sampling in the Northwest Atlantic is less complete but extends across the Labrador Sea to the Grand Banks, the Scotia Shelf, and the Gulf of Maine.
The copepod Calanus finmarchicus contributes >50% of the biomass of sampled plankton in the North Atlantic. Its population has declined substantially in the Northeast Atlantic since the early 1960s (Fig. 9.25), apparently as a function of variation in the North Atlantic Oscillation (NAO)[5]. Also, recent and persistent declines seem to be related to a low-frequency change in the volume of Norwegian Sea Deep Water, where Calanus finmarchicus overwinters[6]. Figure 9.26 shows that, in contrast, the arctic species Calanus glacialis extended its range in the Northwest Atlantic during the 1990s as a consequence of the extension of cold Labrador Slope Water[5].
Historical time series of zooplankton biomass suggest a decrease in biomass between 1954 and 1995 in the oceanic and outer shelf regions of the eastern Bering Sea[7]. However, when the data are separated by shelf region, such a trend is not apparent[8]. Inshore sampling of Calanus marshallae indicates a much higher biomass in the late 1990s compared to the early 1980s. Water temperature is the most important factor influencing zooplankton growth rates and may be responsible for the observed interannual variability in mid-shelf zooplankton biomass[9]. During cold springs when the spring bloom is dominated by ice-edge blooms, reduced coupling between the mesozooplankton and phytoplankton means more phytoplankton will be ungrazed and sink to the bottom, so enhancing the benthic food web. Stronger coupling between mesozooplankton and phytoplankton in warmer springs may result in a stronger pelagic production.
The population of the jellyfish Chrysaora melamaster increased at least ten-fold during the 1990s[10]. These large jellyfish compete for food with young walleye pollock (consuming an estimated 5% of the annual crop of zooplankton) and also feed upon them (consuming an estimated 3% of newborn walleye pollock). Jellyfish have very low energy requirements compared with fish (20 times less) and mammals (200 times less than whales) on a per unit weight basis. Their increased abundance may be due to reduced nutrients and a lower-energy plankton regime.
Benthos (9.3.3.2)
Data are available for sedentary and long-lived macrozoobenthos, which are relevant indicators of multi-year environmental fluctuations between the late 1700s and the present. Biogeographical boundaries in the Barents Sea have shifted as a result of temperature fluctuations[11]. Based on analyses using temperature paleo-reconstructions, it appears that high arctic species tend to survive only when temperatures remain between -1.8 and 6°C, whereas adults of boreal species can survive temperatures of -1 to 25°C. Also, biogeographical changes in the bottom fauna appear to occur faster and are more easily detected during warm periods than cold periods.
The zoobenthos of the Russian Arctic seas has been most intensively studied in the Barents Sea. Deryugin[12] detected several unusual species in Kola Bay in 1908 and 1909 and related this to fluctuations in water temperature. Some boreal species in the Barents Sea have responded to environmental change by shifting their biogeographical borders[13] (Fig. 9.27). This reflects variations in population size at habitat boundaries, not changes in the size and shape of the habitats themselves[14].
In years following warming, the polychaete Spiochaetopterus typicus predominates along the Kola Section in the Barents Sea. Following cold years, the polychaete Maldane sarsi predominates. Spiochaetopterus typicus is thus an indicator of warming or warm conditions.
Estimating natural fluctuations in zoobenthos biomass in the Barents Sea is difficult owing to the impact of commercial bottom trawling[15]. In the Pechora Sea, where there is no traditional demersal fishery, changes in zoobenthos biomass in 1924, 1958 to 1959, 1968 to 1970, and 1992 to 1995 show a negative correlation between zoobenthos biomass and temperature[16].
In the Bering Sea, long-term change in zoobenthos communities is known for the eastern regions as a result of Soviet and American investigations in 1958 to 1959 and 1975 to 1976. In the 1950s, maximum biomass occurred in the northwestern part of the eastern Bering Sea in the mid-shelf region at bottom depths between 50 and 150 m. In the early 1970s, the highest biomasses occurred in the mid-shelf area, southeast of the Pribilof Islands. Because the early 1970s were cold compared to the late 1950s, it may be that the difference in zoobenthos biomass related to changes in the southern limit of the ice edge and thus to the amount of ice-edge primary production that fell to the benthos, ungrazed by pelagic zooplankton.
Recent studies indicate ongoing change in the benthic communities of the Bering and Chukchi Seas[17]. The region just north of the Bering Strait is a settling basin for organic carbon, which results in a high benthic standing stock and high oxygen uptake rates[18].
Benthic productivity in this region near 67°30’ N, 169° W has historically maintained the highest benthic faunal biomass of the entire Bering/Chukchi system[19]. Although benthic biomass remains high in the area, regional changes in the dominant benthic species have occurred. This is likely to indicate changing hydrographic conditions[20].
In the St. Lawrence Island polynya region, changes in regional oceanography due to the position or size of the Gulf of Anadyr gyre are ultimately related to the northward transport of water through the Bering Strait, and to the geostrophic balance within the Arctic Ocean basin. The latter, which is related to variations in the NAO/AO index, drives the northward current system in the northern Bering Sea[21]. Roach et al.[22] found little flow through the Bering Strait into the Arctic Ocean during the NAO-positive period of the early 1990s, and a large increase in flow when the NAO became negative in 1996. Small flow into the Arctic Ocean is coincident with reduced northward transport of water south of St. Lawrence Island.
The Gulf of Anadyr "cold pool" is maintained by sea-ice production and brine formation in the St. Lawrence Island polynya. Reduced sea-ice production to the south of the polynya resulting in a decreased supply of nutrients for early-season primary production would limit benthic populations[23]. However, it is possible that an enhanced and more energetic polynya could result from warming. This could maintain a chemostat-type bloom system, as to the north of St. Lawrence Island[24], allowing a longer growing season and greater production and thus transport.
The three species of crab that inhabit the eastern Bering Sea shelf (red king crab,Tanner crab, and snow crab) exhibit highly periodic patterns in abundance. Rosenkranz et al.[25] found that anomalously cold bottom [[temperature]s] in Bristol Bay may adversely affect the reproductive cycle of Tanner crab and that northeasterly winds may promote coastal upwelling, which advects larvae to regions of fine sediments favorable for survival upon settling. Incze and Paul[26] linked low densities of copepods within the 70 meter (m) isobath in Bristol Bay with low abundance of Tanner crab larvae. Recruitment patterns for red king crab in Bristol Bay show the populations to be negatively correlated with the deepening of the Aleutian Low and warmer water temperatures[27]. Red king crabs were commercially exploited during the late 1970s, which has also contributed to the population decline.
Fish (9.3.3.3)
There are few records of marine biota showing interannual and longer-term variability in the Arctic Ocean, but records of the abundance of commercial fish species for the Labrador, Greenland, Iceland, Norwegian, and Barents Sea go back to the start of the twentieth century and even earlier in some cases. Within these areas capelin, cod, and herring populations have undergone very large fluctuations in biomass and distribution.
The period of warming from the mid-1920s to the mid-1960s, which affected Greenland and Iceland in particular (see section 9.2.4.2 (Past variability in Arctic marine systems)), had a profound effect on the major commercial fish species and also on most other marine life. A number of species, which had been rare in offshore areas west of Greenland, became abundant at this time and population biomass increased by several orders of magnitude. These changes were not related to fishing and are clearly due to climate variability.
Boreal species such as cod are likely to respond strongly to temperature variability and so show greater variability in recruitment at the extremes of their range[28]. However, for the period over which records are available most populations have been reduced to low levels as a result of fishing pressure and may therefore show high variability throughout their distribution.
The warming period of the 1920s
The warming period of the 1920s caused a poleward extension in the range of distribution for many fish and other marine and terrestrial species from Greenland to Iceland and eastward to the Kara Sea. Records of changes in species distribution during the 1920s provide some of the most convincing evidence of the pervasive effects of a change in climate on the marine ecosystem as a whole. Jensen[29] published a comprehensive review of the effects of the climate change in the Arctic and subarctic regions during this period, which presents much of this information. Some of the salient points concerning fish species are summarized in Table 9.9.
The marine shelf ecosystem off West Greenland is affected by cold polar water masses and temperate Atlantic water. Changes in the distribution of these water masses, under the influence of the NAO, affect the distribution and abundance of fish species and hence fisheries yields[30].
The distribution of cod extended poleward by about 1,000 kilometers (km) between 1920 and 1930 and can be followed in some detail, because fishing stations were established progressively further north as directed coastal fisheries were established by the Greenland Administration.
The international offshore fishery for cod off West Greenland reached a peak of over 400,000 tonnes in the early 1960s before collapsing. The decline was due to a combination of fishing pressure and reduced water temperature, and probably to a lack of recruitment from Iceland. The relationship between water temperature and recruitment level is clear for this area[31], with poor recruitment occurring at temperatures below about 1.5°C (measured on Fylla Bank, 64° N, in June). The warming of the North Atlantic that has taken place since about the early 1990s has also affected Greenland but temperatures remained below 1.5°C until 1996. Thus it is too early to expect a recovery of the cod population; cod take about seven years to reach maturity and may be adversely affected by the trawl fishery for shrimps which is now the mainstay of the Greenland fisheries.
Table 9.9. Changes in the distribution and abundance of fish species off West Greenland and Iceland during the period of warming from 1920 onwards. Prepared by Brander[32] based on Saemundsson[33] and Jensen[34]. | ||
West Greenland |
Iceland | |
Species previously absent, but which appeared from 1920 onwards |
Haddock (Melanogrammus |
Bluntnose six-gill shark (Notidanus griseus), swordfish (Xiphias gladius), horse mackerel (Trachurus trachurus) |
Rare species which became more common and extended their ranges |
Coal fish (Pollachius virens; new ecords of spawning fish), Atlantic salmon (Salmo salar), spurdog (Squalus acanthias) |
Witch (Glyptocephalus cynoglossus), turbot (Psetta maxima), basking shark (Cetorhinus maximus), northern bluefin tuna (Thunnus thynnus), mackerel (Scomber scombrus),Atlantic saury (Scomberesox saurus), ocean sunfish (Mola mola) |
Species which became abundant and extended their ranges poleward |
Atlantic cod, Atlantic herring (new records of spawning fish) |
Atlantic cod, Atlantic herring (both extended their spawning distribution) |
Arctic species which no longer occurred in southern areas, and extended their northern limits |
Capelin, Greenland cod, Greenland halibut (became much less common) |
Capelin |
One of the principal changes which took place off Iceland during the 1920s warming period occurred within the major pelagic populations – those of herring and capelin (Table 9.9). Prior to 1920, the capelin spawned regularly on the south and southwest coasts of Iceland, but from 1928 to 1935 very few capelin were taken in these areas. In contrast, herring extended their spawning areas from the south and southwest coast to the east, northwest, and north coasts in this period[35].
Similar changes are also recorded for Jan Mayen, the Barents Sea, the Murman Coast, the White Sea, Novaya Zemlya, and the Kara Sea, where cod and herring extended their ranges and became more abundant.
Climate effects on fish in the Barents Sea
Understanding of the processes underlying major fluctuations in the fish ecosystem of the Barents Sea is considerably better than for most other areas of the northern North Atlantic[36]. The main species involved are Atlantic cod, capelin, and Atlantic herring. These species are closely linked through the food web. Cod is highly dependent on capelin as its main prey. One- to two-year old herring prey heavily on the larvae of capelin, whose mortality increases greatly in years with a large biomass of young herring. Interactions between these species are strongly affected by the highly variable oceanographic conditions of the Barents Sea[37].
The early years of the twentieth century, particularly 1902, were extremely cold in the Barents Sea, with extensive sea-ice cover. This resulted in a crisis for the Norwegian fisheries, with low catches of small Northeast Arctic cod in very poor condition. Large numbers of seals, primarily harp seals, moved down the Norwegian coast from the Barents Sea. A similar sequence of events occurred during the cold period in the 1980s, when the capelin population collapsed; the cod were small and in poor condition and harp seals again invaded the northern coast of Norway.
For cod in particular, the consequences of variability in water temperature, transport, and food during early life stages have been studied closely[38]. Growth and survival rates of larvae and juveniles are higher in warm years and the large year-classes of cod spread further east into the Barents Sea, where they encounter cooler water and their growth rate slows as a result[39].
Norwegian spring-spawning herring
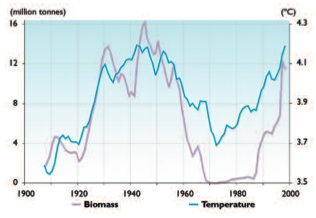
The biomass of Norwegian spring-spawning herring increased almost ten-fold between 1920 and 1930, when the Norwegian Sea and much of the North Atlantic went through a period of rapid warming[41]. The herring population declined rapidly from the late 1950s and by 1970 had decreased by more than four orders of magnitude. The decline was coincident with a period of cooling (Fig. 9.28). Although this cooling may have been a contributing factor, it is likely that heavy fishing pressure was the primary cause of the collapse of the population.
The collapse of the Norwegian spring-spawning herring population coincided with a retraction of the summer feeding distribution due to the southward and eastward shift in the location of the Polar Front. The Polar Front was to the north of Iceland prior to 1965 but has since stayed west of Iceland. Despite a complete recovery of the herring spawning stock and a rise in water temperature north of Iceland in 2000 to levels similar to those of the mid-1960s[42], the herring have not returned to their earlier feeding areas.
The rapid cooling during the mid- and late 1960s also resulted in reduced growth and recruitment of the Norwegian spring-spawning herring[43]. The same happened with the Icelandic summer spawning herring[44].
Temperature-mediated habitat changes in Canadian capelin
Capelin off Newfoundland and Labrador spread southward as far as the Bay of Fundy when water temperatures declined south of Newfoundland in the mid-1960s and retracted northward as water temperatures rose in the 1970s[45]. During cooling in the latter half of the 1980s and into the 1990s, capelin again extended their range, eastward to Flemish Cap and southward onto the northeastern Scotia Shelf off Nova Scotia[46]. For example, small quantities of capelin began to appear in the groundfish trawl surveys on the Scotia Shelf in the mid-1980s and since then numbers have increased dramatically[47]. Initially, only adult capelin were caught, but juveniles later appeared, suggesting capelin were successfully spawning.
This shift appears to have been part of a larger-scale ecosystem change. While capelin were spreading onto the Scotia Shelf, polar cod, whose primary grounds have traditionally been the Labrador Shelf stretching southward to northern Newfoundland, were moving southward. In the late 1980s and early 1990s, as water temperatures decreased, polar cod pushed southward onto the Grand Banks and into the Gulf of St. Lawrence in large numbers[48].
Historical climate and fish in the Bering Sea
The direct effects of atmospheric forcing resulting from climate variations are very important to the physical oceanographic conditions of the Bering Sea. Since the eastern Bering Sea shelf has a characteristically sluggish mean flow and is separated from any direct oceanographic connection to the North Pacific Ocean by the Alaska Peninsula, linkages between the eastern Bering Sea and the climate system are primarily a result of the ocean–atmosphere interaction[49]. Climate variations in this region are directly linked to the location and intensity of the Aleutian Low pressure center, which affects winds, surface heat fluxes, and the formation of sea ice[50]. The pressure index has experienced eight statistically significant shifts on roughly decadal time scales that alternated between cool and warm periods[51]. A well-documented shift[52] from a cool to a warm period occurred between 1977 and 1989, which coincided with the start of fishery-independent sampling programs and fishery catch monitoring of major groundfish species. Information from the contrast between this period and the previous and subsequent cool periods (1960 to 1976 and 1989 to 2000) forms the basis of the following discussion.
Changes in atmospheric climate are primarily transmitted through the Bering Sea to the biota via the mechanisms of wind stress[53] and the annual variation in sea ice extent[54]. These mechanisms directly alter the timing and abundance of primary and secondary production by changing the salinity, mixed-layer depth, nutrient supply, and vertical mixing in the ocean system.
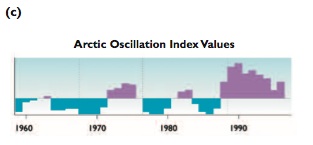
The extent and timing of the sea ice also determines the area where cold bottom water [[temperature]s] will persist throughout the following spring and summer. This eastern Bering Sea area of cold water, known as the cold pool, varies with the annual extent and duration of the ice pack and can influence fish distributions. Pollock have shown a preference for warmer water, and exhibit an avoidance of the cold pool[56]. In cold years they utilize a smaller portion of the shelf waters, in contrast to warm years when they have been observed as far north as the Bering Strait and the Chukchi Sea. Strong year-classes of pollock have been found to occur synchronously throughout the Bering Sea[57] and to coincide with above-normal air and bottom water temperatures and reduced sea-ice cover[58]. These favorable years of production are the result of good juvenile survival and are related to how much cold water habitat is present[59], the distribution of juveniles relative to the adult population which influences the level of predation[60], and enhanced rates of embryonic development in warmer water[61].
The distributions of forage fishes including Pacific herring, capelin, eulachon (Thaleichthys pacificus), and juvenile Pacific cod and pollock indicate temperature-related differences[62]. Capelin exhibits an expanded range in years with a larger cold pool and contracts in years of reduced sea-ice cover. Although the productivity of capelin populations in relation to water temperature is not known, Bering Sea herring populations exhibited improved recruitment during warm years[63], similar to other herring populations where the timing of spawning is also temperature-related[64].
Recruitment and stock biomass have been examined for evidence that climatic shifts induce responses in the production of groundfish species in the Bering Sea and North Pacific Ocean[65]. Even though results from these studies are highly variable, strong autocorrelation in recruitment associated with the significant change in climate in 1977 was observed for many salmonids and some winter-spawning flatfish species such as eastern Bering Sea arrowtooth flounder and Greenland halibut. The two latter species showed opposite changes post-1977 (increasing biomass for arrowtooth flounder and decreasing biomass for Greenland halibut). Substantial increases in the abundance of Pacific cod, skates, flatfish such as rock sole, and non-crab benthic invertebrates also took place on the Bering Shelf in the 1980s[66].
The decadal-scale patterns in recruitment success for winter-spawning flatfish (Fig. 9.29) may be associated with decadal shifts in the Aleutian low pressure system that affects cross-shelf advection patterns of larvae to favorable nursery areas rather than with water temperature[67].
Marine mammals and seabirds (9.3.3.4)
Although fragmented, there is a lot of evidence to suggest that climate variations have profound effects on marine mammals and seabirds. The capelin collapse in the Barents Sea in 1987 had a devastating effect on seabirds breeding on Bjørnøya. Repeated years (1967, 1981, 2000, 2001, and 2002) with little or no sea ice in the Gulf of St. Lawrence resulted in years with almost zero production of seal pups, compared to hundreds of thousands in good sea-ice years.
Vibe[68] explored the relationship between climate fluctuations and the abundance and distribution of animals, including marine mammals and seabirds, in Greenland. During the cold, dry, and stable "drift-ice stagnation" phase in West Greenland (approximately 1810 to 1860), marine mammals and seabirds concentrated at central West Greenland because the sea ice did not advance far north into Davis Strait. During the "drift-ice pulsation stage" (1860 to 1910), when the sea ice of the Arctic Ocean drifted into the Atlantic Ocean in larger amounts than before, marine mammal and seabird [[population]s] decreased in the unstable and wet climate of West Greenland because the East Greenland Current and the East Greenland sea ice advanced far north into Davis Strait in summer. In the same period, the Greenland right whale (Balaena mysticetus) population "stagnated" in the Atlantic region. During the "drift-ice melting stage" (1910 to 1960) the East Greenland sea ice decreased in Davis Strait and populations of marine mammals and seabirds increased in northern West Greenland. Cod were abundant along the coast of West Greenland and multiplied in Greenland waters.
The condition of adult male and female polar bears has declined in Hudson Bay since the early 1980s, as have birth rates and the proportion of first-year cubs in the population. Stirling et al.[69] suggest that the proximate cause of these changes in physical and reproductive parameters is a trend toward earlier breakup of the sea-ice, which has resulted in the bears coming ashore in poorer condition.
Chapter 9: Marine Systems
9.1. Introduction (Past variability in Arctic marine systems)
9.2. Physical oceanography
9.2.1. General features (Past variability in Arctic marine systems)
9.2.2. Sea ice (Sea ice effect on marine systems in the Arctic)
9.2.3. Ocean processes of climatic importance
9.2.4. Variability in hydrographic properties and currents
9.2.5. Anticipated changes in physical conditions
9.3. Biota
9.3.1. General description of the Arctic biota community
9.3.2. Physical factors mediating ecological change
9.3.3. Past variability – interannual to decadal
9.3.4. Future change – processes and impacts on biota
9.4. Effects of changes in ultraviolet radiation
9.5. The carbon cycle and climate change
9.6. Key findings (Past variability in Arctic marine systems)
9.7. Gaps in knowledge and research needs
References
Citation
Committee, I. (2012). Past variability in Arctic marine systems. Retrieved from http://editors.eol.org/eoearth/wiki/Past_variability_in_Arctic_marine_systems- ↑ Thordardóttir, T., 1984. Primary production north of Iceland in relation to water masses in May-June 1970?1989. ICES CM 1984/L:20. International Council for the Exploration of the Sea, Copenhagen, 17pp.
- ↑ Johnsen, G., Z. Volent, K. Tangen and E. Sakshaug, 1997. Time series of harmful and benign phytoplankton blooms in northwest European waters using the Seawatch buoy system. In: M. Kahru and C.W. Brown, (eds.). Monitoring Algal Blooms: New Techniques for Detecting Large-scale Environmental Change, pp. 115?143. Landes Bioscience.
- ↑ Dahl, E., B. Edvardsen and W. Eikrem, 1998. Chrysochromulina blooms in the Skagerrak after 1988. In: B. Reguera, J. Blanco, M.L. Fernández and T. Wyatt (eds.). Harmful Microalgae, pp. 104?105. Intergovernmental Oceanographic Commission.–Dahl, E.,T. Aune and K. Tangen, 2001. Shellfish toxicity in Norway ? experiences from regular monitoring, 1992?1999. In: G.M. Hallegraeff, S.I. Blackburn, C.J. Bolch and R.J. Lewis (eds.). Harmful Algal Blooms 2000, pp. 425?428. Intergovernmental Oceanographic Commission
- ↑ Johns, D.G., 2001. Calanus abundance in the North Atlantic as determined from CPR-surveys. In: Proceedings of the workshop on The Northwest Atlantic Ecosystem - a Basin Scale Approach, pp. 44?47. Canadian Science Advisory Secretariat, Proceedings Series 2001/23.
- ↑ 5.0 5.1 Heath, M.R., J.O. Backhaus, K. Richardson, E. McKenzie, D. Slagstad, D. Beare, J. Dunn, J.G. Fraser, A. Gallego, D. Hainbucher, S. Hay, S. Jonasdottir, H. Madden, J. Mardaljevic and A. Schacht, 1999. Climate fluctuations and the spring invasion of the North Sea by Calanus finmarchicus. Fisheries Oceanography 8 (suppl.1):163?176.
- ↑ Planque, B. and S.D. Batten, 2000. Calanus finmarchicus in the North Atlantic: the year of Calanus in the context of interdecadal change. ICES Journal of Marine Science, 57(6):1528?1535.
- ↑ Johns, D.G., 2001. Calanus abundance in the North Atlantic as determined from CPR-surveys. In: Proceedings of the workshop on The Northwest Atlantic Ecosystem - a Basin Scale Approach, pp. 44?47. Canadian Science Advisory Secretariat, Proceedings Series 2001/23.
- ↑ Sugimoto, T. and K. Tadokoro, 1998. Interdecadal variations of plankton biomass and physical environment in the North Pacific. Fisheries Oceanography, 7(3?4):289?299.
- ↑ Napp, J.M., C.T. Baier, R.D. Brodeur, K.O. Coyle, N. Shiga, and K. Mier, 2002. Interannual and decadal variability in zooplankton communities of the southeastern Bering Sea shelf. Deep-Sea Research II, 49:5991?6008.
- ↑ Coyle, K.O. and A.I. Pinchuk, 2002. Climate-related differences in zooplankton density and growth on the inner shelf of the southeastern Bering Sea. Progress in Oceanography, 55:177?194.–Napp, J.M., A.W. Kendall Jr. and J.D. Schumacher, 2000. A synthesis of biological and physical processes affecting the feeding environment of larval walleye pollock (Theragra chalcogramma) in the eastern Bering Sea. Fisheries Oceanography, 9:147?162.
- ↑ Brodeur, R.D., C.E. Mills, J.E. Overland, G.E. Walters and J.D. Schumacher, 1999b. Evidence for a substantial increase in gelatinous zooplankton in the Bering Sea, with possible links to climate change. Fisheries Oceanography, 8:296?306.
- ↑ Blacker, R.W., 1965. Recent changes in the benthos of the West Spitzbergen fishing grounds. International Commission for the Northwest Atlantic Fisheries, Special Publication, 6:791?794.
- ↑ Deryugin, K.M., 1924. The Barents Sea at the Kola Meridian (33°30E). Trudy Severnoi Naucho-Promyslovoi Ekspeditsii, 19:3?103. (In Russian)
- ↑ Chemerisina, V.T., 1948. On zoogeography of the Barents Sea. Trudy Murmanskoy Biologicheskoy Stantzii AN SSSR, 1:293?298. (In Russian)–Nesis, K.N., 1960. Fluctuations of the Barents Sea bottom fauna under the effect of the hydrobiological regime variation (at the Kola Meridian transect). Sovetskie rybokhozyaystvennye issledovania v moryakh Evropeyskogo Severa. Moscow, pp. 129?137. (In Russian)
- ↑ Galkin, Yu.I., 1998. Long-term changes in the distribution of molluscs in the Barents Sea related to the climate. Berichte zur Polarforschung, 287:100?143.
- ↑ Denisenko, S.G., 2001. Long-term changes of zoobenthos biomass in the Barents Sea. Proceedings of the Zoological Institute of the Russian Academy of Sciences, 289:59?66.
- ↑ Denisenko, S.G., 2003. pers. comm., Zoological Institute RAS, St. Petersburg.
- ↑ Francis, R.C., S.R. Hare, A.B. Hollowed and W.S. Wooster, 1998. Effects of interdecadal climate variability on the oceanic ecosystems of the NE Pacific. Fisheries Oceanography, 7:1?21.–Grebmeier, J.M. and L.W. Cooper, 1995. Influence of the St Lawrence Island polynya upon the Bering Sea benthos. Journal of Geophysical Research ? Oceans, 100(C3):4439?4460.–Sirenko, B.I. and V.M. Koltun, 1992. Characteristics of benthic biocenoses of the Chukchi and Bering Seas. In: P.A. Nagel (ed.). Results of the Third Joint US-USSR Bering and Chukchi Seas Expedition (BERPAC), Summer 1988, pp 251?258. U.S. Fish and Wildlife Service,Washington, D.C.
- ↑ Grebmeier, J.M., 1993. Studies of pelagic-benthic coupling extended onto the Soviet continental shelf in the northern Bering and Chukchi Seas. Continental Shelf Research, 13(5?6):653?668.–Grebmeier, J.M., C.P. McRoy and H.M. Feder, 1988. Pelagic-benthic coupling on the shelf of the northern Bering and Chukchi Seas. 1. Food supply source and benthic biomass. Marine Ecology Progress Series, 48(1):57-67.–Grebmeier, J.M., H.M. Feder and C.P. McRoy, 1989. Pelagic-benthic coupling on the shelf of the northern Bering and Chukchi Seas. 2. Benthic community structure. Marine Ecology Progress Series, 51(3):253?268.
- ↑ Grebmeier, J.M., 1993. Studies of pelagic-benthic coupling extended onto the Soviet continental shelf in the northern Bering and Chukchi Seas. Continental Shelf Research, 13(5?6):653?668.–Grebmeier, J.M. and L.W. Cooper, 1994. A decade of benthic research on the continental shelves of the northern Bering and Chukchi seas: Lessons learned. In: R.H. Meehan, V. Sergienko and G. Weller (eds.). Bridges of Science Between North America and the Russian Far East, pp. 87?98. American Association for the Advancement of Science, Arctic Division, Fairbanks, Alaska.–Grebmeier, J.M., W.O. Smith and R.J. Conover, 1995. Biological processes on Arctic continental shelves: ice-ocean-biotic interactions. In: W.O. Smith and J.M. Grebmeier (eds.). Arctic Oceanography: Marginal Zones and Continental Shelves. Coastal and Estuarine Studies, 49:231?261.–Stoker, S.W., 1978. Benthic Invertebrate Macrofauna of the Eastern Continental Shelf of the Bering and Chukchi Seas. Ph.D. Thesis, University of Alaska, Fairbanks, 155pp.+ app.–Stoker, S.W., 1981. Benthic invertebrate macrofauna of the eastern Bering/Chukchi continental shelf. In:W. Hood and J.A. Calder (eds.). The Eastern Bering Sea Shelf: Oceanography and Resources. Vol. 2, pp. 1069?1090. University of Washington Press, Seattle.
- ↑ Grebmeier, J.M., 1993. Studies of pelagic-benthic coupling extended onto the Soviet continental shelf in the northern Bering and Chukchi Seas. Continental Shelf Research, 13(5?6):653?668.–Grebmeier, J.M., W.O. Smith and R.J. Conover, 1995. Biological processes on Arctic continental shelves: ice-ocean-biotic interactions. In: W.O. Smith and J.M. Grebmeier (eds.). Arctic Oceanography: Marginal Zones and Continental Shelves. Coastal and Estuarine Studies, 49:231?261.
- ↑ Walsh, J.J., C.P. McRoy, L.K. Coachman, J.J. Goering, J.J. Nihoul, T.E. Whitledge, T.H. Blackburn, P.L. Parker, C.D. Wirick, P.G. Stuert, J.M. Grebmeier, A.M. Springer, R.D. Tripp, D.A. Hansell, S. Djenidi, E. Deleersnijder, K. Henriksen, B.A. Lund, P. Andersen, F.E. Muller-Karger and K. Dean, 1989. Carbon and nitrogen cycling within the Bering/Chukchi Seas: Source regions for organic matter effecting AOU demands of the Arctic Ocean. Progress in Oceanography, 22:277?359.
- ↑ Roach, A.T., K. Aagaard, C.H. Pease, S.A. Salo,T. Weingartner, V. Pavlov and M. Kulakov, 1995. Direct measurements of transport and water properties through the Bering Strait. Journal of Geophysical Research, 100(C9):18443?18458.
- ↑ Grebmeier, J.M. and L.W. Cooper, 1995. Influence of the St Lawrence Island polynya upon the Bering Sea benthos. Journal of Geophysical Research ? Oceans, 100(C3):4439?4460.
- ↑ Walsh, J.J., C.P. McRoy, L.K. Coachman, J.J. Goering, J.J. Nihoul, T.E. Whitledge, T.H. Blackburn, P.L. Parker, C.D. Wirick, P.G. Stuert, J.M. Grebmeier, A.M. Springer, R.D. Tripp, D.A. Hansell, S. Djenidi, E. Deleersnijder, K. Henriksen, B.A. Lund, P. Andersen, F.E. Muller-Karger and K. Dean, 1989. Carbon and nitrogen cycling within the Bering/Chukchi Seas: Source regions for organic matter effecting AOU demands of the Arctic Ocean. Progress in Oceanography, 22:277?359.
- ↑ Rosenkranz, G.E., A.V. Tyler and G.H. Kruse, 2001. Effects of water temperature and wind on year-class success of Tanner crabs in Bristol Bay, Alaska. Fisheries Oceanography, 10:1?12.
- ↑ Incze, L.S. and A.J. Paul, 1983. Grazing and predation as related to energy needs of stage I zoeae of the Tanner crab Chinoectes bairdi (Brachyura, Majidae). Biological Bulletin, 165:197?208.
- ↑ Zheng, J. and G.H. Kruse, 2000. Recruitment patterns of Alaskan crabs in relation to decadal shifts in climate and physical oceanography. ICES Journal of Marine Science, 57:438?451.
- ↑ Brander, K., 2000. Effects of environmental variability on growth and recruitment in cod (Gadus morhua) using a comparative approach. Oceanologica Acta, 23(4):485?496.–Ottersen, G. and N.C. Stenseth, 2001. Atlantic climate governs oceanographic and ecological variability in the Barents Sea. Limnology and Oceanography, 46:1774?1780.
- ↑ Jensen, A.S., 1939. Concerning a change of climate during recent decades in the Arctic and subarctic regions, from Greenland in the west to Eurasia in the east, and contemporary biological and geophysical changes. Biologiske Meddelelser 14, 75pp.
- ↑ Pedersen, S.A. and J.C. Rice, 2002. Dynamics of fish larvae, zooplankton, and hydrographical characteristics in the West Greenland large marine ecosystem 1950?1984. In: K. Sherman and H.R. Skjoldal (eds.). Large Marine Ecosystems of the North Atlantic, pp. 151?193. Elsevier.–Pedersen, S.A. and E.L.B. Smidt, 2000. Zooplankton distribution and abundance in West Greenland waters, 1950?1984. Journal of Northwest Atlantic Fishery Science, 26:45?102.–Schopka, S.A., 1994. Fluctuations in the cod stock off Iceland during the twentieth century in relation to changes in the fisheries and environment. ICES Marine Science Symposia, 198:175?193.
- ↑ Brander, K., 2000. Effects of environmental variability on growth and recruitment in cod (Gadus morhua) using a comparative approach. Oceanologica Acta, 23(4):485?496.–Buch, E., S.A. Horsted and H. Hovgård, 1994. Fluctuations in the occurrence of cod in the Greenland waters and their possible causes. ICES Marine Science Symposia, 198:158?174.
- ↑ Brander, K., 2003. Fisheries and climate. In: G. Wefer, F. Lamy and F. Mantoura (eds.). Marine Science Frontiers for Europe, pp 29?38. Springer-Verlag.
- ↑ Saemundsson B., 1937. Fiskirannsoknir. Andvari, vol. 62 Reykjavik. (In Icelandic)
- ↑ Jensen, A.S., 1939. Concerning a change of climate during recent decades in the Arctic and subarctic regions, from Greenland in the west to Eurasia in the east, and contemporary biological and geophysical changes. Biologiske Meddelelser 14, 75pp.
- ↑ Saemundsson B., 1937. Fiskirannsoknir. Andvari, vol. 62 Reykjavik. (In Icelandic)
- ↑ Rødseth,T., 1998. Models for Multispecies Management. Springer-Verlag, 246pp.
- ↑ Hamre, J., 1994. Biodiversity and exploitation of the main fish stocks in the Norwegian-Barents Sea ecosystem. Biodiversity and Conservation, 3:473?492.
- ↑ Michalsen, K., G. Ottersen and O. Nakken, 1998. Growth of North-east Arctic cod (Gadus morhua L.) in relation to ambient temperature. ICES Journal of Marine Science, 55:863?877.–Ottersen, G. and H. Loeng, 2000. Covariability in early growth and year-class strength of Barents Sea cod, haddock and herring: The environmental link. ICES Journal of Marine Science, 57:339?348.–Ottersen, G., K. Michalsen and O. Nakken, 1998. Ambient temperature and distribution of north-east Arctic cod. ICES Journal of Marine Science, 55:67?85.–Sætersdal, G. and H. Loeng, 1987. Ecological adaption of reproduction in Northeast Arctic cod. Fisheries Research, 5:253?270.
- ↑ Toresen, R. and O.J. Østvedt, 2000. Variation in abundance of Norwegian spring-spawning herring (Clupea harengus, Clupeidae) throughout the 20th century and the influence of climatic fluctuations. Fish and Fisheries, 1:231?256.
- ↑ Ottersen, G., H. Loeng, B. Adlandsvik and R. Ingvaldsen, 2003. Temperature variability in the Northeast Atlantic. ICES Marine Science Symposia, 219:86?94.
- ↑ Toresen, R. and O.J. Østvedt, 2000. Variation in abundance of Norwegian spring-spawning herring (Clupea harengus, Clupeidae) throughout the 20th century and the influence of climatic fluctuations. Fish and Fisheries, 1:231?256.
- ↑ Malmberg, S.-A. and H. Valdimarsson, 2003. Hydrographic conditions in Icelandic waters, 1990?1999. ICES Marine Science Symposia, 19:50?60.
- ↑ Toresen, R. and O.J. Østvedt, 2000. Variation in abundance of Norwegian spring-spawning herring (Clupea harengus, Clupeidae) throughout the 20th century and the influence of climatic fluctuations. Fish and Fisheries, 1:231?256.
- ↑ Jakobsson, J., A. Gudmundsdottir and G. Stefansson, 1993. Stock-related changes in biological parameters of the Icelandic summer-spawning herring. Fisheries Oceanography, 2(3?4):260?277.
- ↑ Colton, J.B. Jr., 1972. Temperature trends and the distribution of groundfish in continental shelf waters, Nova Scotia to Long Island. Fishery Bulletin, 70:637?657.–Frank, K.T., J. Carscadden and J.E. Simon, 1996. Recent excursions of capelin (Mallotus villosus) to the Scotian Shelf and Flemish Cap during anomalous hydrographic conditions. Canadian Journal of Fisheries and Aquatic Sciences, 53:1473?1486.–Tibbo, S.N. and R.D. Humphreys, 1966. An occurrence of capelin (Mallotus villosus) in the Bay of Fundy. Journal of the Fisheries Research Board of Canada, 23:463?467.
- ↑ Frank, K.T., J. Carscadden and J.E. Simon, 1996. Recent excursions of capelin (Mallotus villosus) to the Scotian Shelf and Flemish Cap during anomalous hydrographic conditions. Canadian Journal of Fisheries and Aquatic Sciences, 53:1473?1486.–Nakashima, B.S., 1996. The relationship between oceanographic conditions in the 1990s and changes in spawning behaviour, growth and early life history of capelin (Mallotus villosus). NAFO Scientific Council Studies, 24:55?68.
- ↑ Frank, K.T., J. Carscadden and J.E. Simon, 1996. Recent excursions of capelin (Mallotus villosus) to the Scotian Shelf and Flemish Cap during anomalous hydrographic conditions. Canadian Journal of Fisheries and Aquatic Sciences, 53:1473?1486.
- ↑ Gomes, M.C., R.L. Haedrich and M.G. Villagarcia, 1995. Spatial and temporal changes in the groundfish assemblages on the northeast Newfoundland/Labrador Shelf, northwest Atlantic, 1978?91. Fisheries Oceanography, 4:85?101.–Lilly, G.R., H. Hop, D.E. Stansbury and C.A. Bishop, 1994. Distribution and abundance of polar cod (Boreogadus saida) off southern Labrador and eastern Newfoundland. ICES CM 1994/O:6. International Council for the Exploration of the Sea, Copenhagen, 21pp.
- ↑ Stabeno, P.J., N.A. Bond, N.B. Kachel, S.A. Salo and J.D. Schumacher, 2001. On the temporal variability of the physical environment over the south-eastern Bering Sea. Fisheries Oceanography, 10(1):81?98.
- ↑ Overland, J.E., J.M. Adams and N.A. Bond, 1999a. Decadal variability of the Aleutian Low and its relation to high latitude circulation. Journal of Climate, 12:1542?1548.
- ↑ Trenberth, K.E., 1990. Recent observed interdecadal climate changes in the Northern Hemisphere. Bulletin of the American Meteorological Society, 71:988?993.
- ↑ Francis, R.C., S.R. Hare, A.B. Hollowed and W.S. Wooster, 1998. Effects of interdecadal climate variability on the oceanic ecosystems of the NE Pacific. Fisheries Oceanography, 7:1?21.
- ↑ Stabeno, P.J., N.A. Bond, N.B. Kachel, S.A. Salo and J.D. Schumacher, 2001. On the temporal variability of the physical environment over the south-eastern Bering Sea. Fisheries Oceanography, 10(1):81?98.
- ↑ Wilderbuer, T.K., A.B. Hollowed, W.J. Ingraham Jr., P.D. Spencer, M.E. Conners, N.A. Bond and G.E. Walters. 2002. Flatfish recruitment response to decadal climate variability and ocean conditions in the eastern Bering Sea. Progress in Oceanography, 55:235?247.
- ↑ Wyllie-Echeverria, T., 1995. Sea-ice conditions and the distribution of walleye pollock (Theragra chalcogramma) on the Bering and Chukchi shelf. In: R.J. Beamish (ed.). Climate Change and Northern Fish Populations. Canadian Special Publication of Fisheries and Aquatic Sciences, 121:131?136.
- ↑ Bulatov, O.A., 1995. Biomass variation of walleye pollock of the Bering Sea in relation to oceanological conditions. In: R.J. Beamish (ed.). Climate Change and Northern Fish Populations. Canadian Special Publication of Fisheries and Aquatic Sciences, 121:631?640.
- ↑ Decker, M.B., G.L. Hunt Jr. and G.V. Byrd Jr., 1995. The relationships among sea surface temperature, the abundance of juvenile walleye pollock, and the reproductive performance and diets of seabirds at the Pribilof Islands, southeastern Bering Sea. In: R.J. Beamish (ed.). Climate Change and Northern Fish Populations. Canadian Special Publication of Fisheries and Aquatic Sciences, 121:425?43–Quinn, T.J. II and H.J. Niebauer, 1995. Relation of eastern Bering Sea walleye pollock (Theragra chalcogramma) recruitment to environmental and oceanographic variables. In: R.J. Beamish (ed.). Climate Change and Northern Fish Populations. Canadian Special Publication of Fisheries and Aquatic Sciences, 121: 497?507.
- ↑ Ohtani, K. and T. Azumaya, 1995. Influence of interannual changes in ocean conditions on the abundance of walleye pollock in the eastern Bering Sea. In: R.J. Beamish (ed.). Climate Change and Northern Fish Populations. Canadian Special Publication of Fisheries and Aquatic Sciences, 121: 87?95.
- ↑ Wespestad, V.G., L.W. Fritz, W.J. Ingraham and B.A. Megrey, 2000. On relationships between cannibalism, climate variability, physical transport, and recruitment success of Bering Sea walleye pollock (Theragra chalcogramma). ICES Journal of Marine Science, 57(2):272?278.
- ↑ Blood, D.M., 2002. Low-temperature incubation of walleye pollock (Theragra chalcogramma) eggs from the southeast Bering Sea shelf and Shelikof Strait, Gulf of Alaska. Deep-Sea Research II, 49:6095?6108.–Haynes, E.G. and G. Ingell, 1983. Effect of temperature on rate of embryonic development of walleye pollock (Theragra chalcogramma). Fishery Bulletin, 81:890?894.
- ↑ Brodeur, R.D., M.T. Wilson, G.E. Walters and I.V. Melnikov, 1999a. Forage fishes in the Bering Sea: distribution, species associations, and biomass trends. In: T.R. Loughlin and K. Ohtani (eds.). Dynamics of the Bering Sea, pp. 509?536. University of Alaska Fairbanks.
- ↑ Williams, E.H. and T.J. Quinn II, 2000. Pacific herring, Clupea pallasi, recruitment in the Bering Sea and north-east Pacific Ocean, II: relationships to environmental variables and implications for forecasting. Fisheries Oceanography, 9:300?315.
- ↑ Zebdi, A. and J.S. Collie, 1995. Effect of climate on herring (Clupea pallasi) population dynamics in the Northeast Pacific Ocean. In: R.J. Beamish (ed.). Climate Change and Northern Fish Populations. Canadian Special Publication of Fisheries and Aquatic Sciences 121:277?290.
- ↑ Hollowed, A.B. and W.S. Wooster, 1995. Decadal-scale variations in the eastern subarctic Pacific: B. Response of northeast Pacific fish stocks. In: R.J. Beamish (ed.). Climate Change and Northern Fish populations. Canadian Special Publication of Fisheries and Aquatic Sciences, 121:373?385.–Hollowed, A.B., S.R. Hare and W.S. Wooster, 2001. Pacific Basin climate variability and patterns of Northeast Pacific marine fish production. Progress in Oceanography, 49:257?282.
- ↑ Conners, M.E., A.B. Hollowed and E. Brown, 2002. Retrospective analysis of Bering Sea bottom trawl surveys: regime shift and ecosystem reorganization. Progress in Oceanography, 55:209?222.
- ↑ Wilderbuer, T.K., A.B. Hollowed, W.J. Ingraham Jr., P.D. Spencer, M.E. Conners, N.A. Bond and G.E. Walters. 2002. Flatfish recruitment response to decadal climate variability and ocean conditions in the eastern Bering Sea. Progress in Oceanography, 55:235?247.
- ↑ Vibe, C., 1967. Arctic Animals in Relation to Climate Fluctuations. Danish Zoogeographical Investigations in Greenland. Meddelelser om Gronland, 170(5):227pp.
- ↑ Stirling, I., N.J. Lunn and J. Iacozza, 1999. Long-term trends in the population ecology of polar bears in western Hudson Bay in relation to climate change. Arctic, 52:294?306.