Influence of the Arctic on global climate
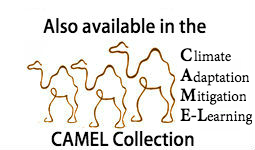
This is Section 2.5 of the Arctic Climate Impact Assessment Lead Author: Gordon McBean. Contributing Authors: Genrikh Alekseev, Deliang Chen, Eirik Førland, John Fyfe, Pavel Y. Groisman, Roger King, Humfrey Melling, Russell Vose, Paul H.Whitfield
Contents
Marine connections (2.5.1)
Although the marine Arctic covers a small fraction of the globe, positive feedback between the Arctic Ocean and the climate system has the potential to cause global effects.The thermohaline circulation is the global-scale overturning in the ocean that transports significant heat via a poleward flow of warm surface water and an equatorward return of cold, less saline water at depth.The overturning crucial to this transport in the Northern Hemisphere occurs in the Greenland, Irminger, and Labrador Seas[1].The occurrence and intensity of overturning is sensitive to the density of water at the surface in these convective gyres, which is in turn sensitive to the outflow of low-salinity water from the Arctic. An increase in arctic outflow is very likely to reduce the overturning and therefore the oceanic flux of heat to northern high latitudes.The overturning also moderates anthropogenic impacts on climate because it removes atmospheric CO2 to the deep ocean. A comprehensive description of the dynamics and consequences of the marine connections is given in Section 9.2.3. (Influence of the Arctic on global climate)
Ice-albedo feedback to warming and cooling (2.5.1.1)
Sea ice is an influential feature of the marine Arctic. It reflects a large fraction of incoming solar radiation and insulates the ocean waters against loss of heat and moisture during winter. Sea ice also inhibits the movement and mixing of the upper ocean in response to wind. By stabilizing the upper ocean through melting, it may control the global heat sink at high northern latitudes [2].
The global impact of ice-albedo feedback is predicated on the existence of a strong relationship between atmospheric temperature increases and sea-ice extent.The seasonal analogue of climate change effects on the marine cryosphere is the dramatic expansion of sea-ice extent in winter and its retreat in summer, in tune with (at a lag of several months) the seasonal variation in air temperature. Another relevant analogue is the seasonal progression from frequently clear skies over the marine cryosphere in winter to dominance by fog and stratiform cloud in summer. The increased moisture supply at the melting surface of the ice pack promotes the formation of low clouds that reflect most of the incoming solar radiation in summer, replacing the weakened reflecting capability of melting sea ice.Thus, cloud cover is an important partner to sea ice in the albedo feedback mechanism.
Freshwater feedback to poleward transport of heat and freshwater (2.5.1.2)
Deep convection in the northwest Atlantic Ocean is a crucial part of the global thermohaline circulation. Water freshened by arctic outflow is cooled, causing it to sink deep into the ocean, from where it flows either south to the North Atlantic or north into the Arctic Basin[3]. Deep convection has considerable interannual variability controlled by atmospheric circulation. It operates to link the stochastic effects of atmospheric variability to slow oscillations in the ocean– atmosphere system via the oceanic transports of heat and “freshwater” in the global thermohaline circulation[4].
The Greenland Sea is one region where new deep water forms[5]. Here, warm and saline water of Atlantic origin meets cold arctic water of lower salinity. Extremely low temperatures cause rapid cooling of the sea surface, which may trigger either deep convective mixing or intensified ice formation, depending on the density of waters at the sea surface. Convection can reach depths of about 2000 m[6] and the temperature change in the water at that depth is an indicator of the intensity of deep-water formation, with warmer temperatures indicating less deep-water formation. Observations show periods of deep-water temperature increases in the Greenland Sea in the late 1950s and between 1980 and 1990, and temperature decreases in the early 1950s and in the late 1960s. A large increase (0.25 ºC) in deepwater temperature occurred in the 1990s[7]. The decrease in deep-water formation implied by increasing deep-water temperatures has weakened the thermohaline circulation, leading to a decreased overflow of deep water through the Faroe- Shetland channel[8].
A reduction in the vertical flux of salt and reduced deep-water formation is likely to trigger a prolonged weakening of the global thermohaline circulation. With less bottom:water formation, there is likely to be a reduction in upwelling at temperate and subtropical latitudes. Paleoclimatic shifts in the thermohaline circulation have caused large and sometimes abrupt changes in regional climate (Section 2.7 (Influence of the Arctic on global climate)). Dickson et al.[9] demonstrated that the flows of dense cold water over sills in the Faroe–Shetland Channel and in Denmark Strait are the principal means of ventilating the deep waters of the North Atlantic. Both the flux and density structure of “freshwater” outflow to the North Atlantic are critical to the arctic influence on global climate via the thermohaline circulation[10].
Sea level (2.5.2)
Global average sea level rose between 0.1 and 0.2 m during the 20th century[11], primarily because of thermal expansion of warming ocean waters. Although the thermal expansion coefficient for seawater is small, integrated over the 6000 m depth of the ocean the resulting change in sea level can generate changes of significance to ecosystems and communities near coastlines.The warming of arctic seawater will have a negligible impact on local sea level because cold (<0 ºC) seawater expands very little with an increase in temperature. However, arctic sea level will respond to changes in the levels of the Atlantic and Pacific Oceans via dynamic links through Bering Strait, Fram Strait, and the Canadian Archipelago. In many areas of the Arctic, sea level is also changing very rapidly as a result of postglacial rebound of the earth’s crust. For example, the land at Churchill, Canada (on the western shore of Hudson Bay), rose one meter during the 20th century. In many parts of the Arctic, changes in the elevation of the shoreline due to crustal rebound are likely to exceed the rise in sea level resulting from oceanic warming.
The Arctic Ocean stores a large volume of “freshwater”. Arctic sea level is sensitive to “freshwater” storage and will rise if this inventory increases, or fall if “freshwater” storage declines. Changes in northern hydrology are therefore likely to have an important effect on arctic sea level by changing “freshwater” storage in the Arctic.
On a timescale of centuries, and with a sufficient increase in temperature, accumulation or ablation of terrestrial ice caps in Greenland and Antarctica are very likely to be the dominant causes of global changes in sea level.There is an interesting aspect to sea-level change in the vicinity of these ice caps: a sea-level increase caused by the ice cap melting, distributed globally, may be offset by changes in the local gravitational anomaly of the ice, which pulls the sea level up towards it. As a result, it is possible that sea level could actually drop at locations within a few hundred kilometers of Greenland, despite an average increase in sea level worldwide. Sections 6.5 (Influence of the Arctic on global climate) and 6.9 (Influence of the Arctic on global climate) provide further details related to ice caps, glaciers, and sea-level rise.
Greenhouse gases (2.5.3)
Arctic ecosystems are characterized by low levels of primary productivity, low element inputs, and slow element cycling due to inhibition of these processes by very cold climatic conditions. However, arctic ecosystems still tend to accumulate organic matter, carbon (C), and other elements because decomposition and mineralization processes are equally inhibited by the cold, wet soil environment[12]. Owing to this slow decomposition, the total carbon (C) and element stocks of wet and moist arctic tundra frequently equal or exceed stocks of the same elements in much more productive ecosystems in temperate and tropical latitudes. Methane (CH4) production, for example, is related to the position of the water table in the active layer, which will be affected by changes in active-layer depth and/or permafrost degradation. Natural gas hydrates are also found in the terrestrial Arctic, although only at depths of several hundred meters. Currently, arctic and alpine tundra is estimated to contain 96 x 1012 kg of C in its soil and permafrost.This is roughly 5% of the world’s soil C pool[13]. An additional 5.7 x 1012 kg of C is stored in arctic wetland, boreal, and tundra vegetation, for a total of 102 x 1012 kg of terrestrial C[14].This is fully discussed in Section 7.4.2.1 (Influence of the Arctic on global climate). Thawing of permafrost has the potential to release large stores of CO2 and CH4 that are presently contained in frozen arctic soils, both as a direct consequence of thawing and as an indirect consequence of changes in soil wetness[15]. Although it is not clear whether the Arctic will be a net source or sink of C in the future, the large amounts of C that could be taken up or released make improved understanding of arctic processes important.
The Arctic Ocean was not initially believed to be a significant sink of C because its sizeable ice cover prevents atmosphere–ocean exchange and biological production in the central ocean was believed to be small. Under warmer climate conditions, however, the amount of C that the Arctic Ocean can sequester is likely to increase significantly. In the northern seas, hydrated CH4 is trapped in solid form at shallow depths in cold sediments. Gas hydrates are likely to decompose and release CH4 to the atmosphere if the temperature of water at the seabed rises by a few degrees[16] over centuries to a millennium. This is discussed further in Section 9.5.5. (Influence of the Arctic on global climate)
Chapter 2: Arctic Climate - Past and Present
2.1 Introduction (Influence of the Arctic on global climate)
2.2 Arctic atmosphere
2.3 Marine Arctic
2.4 Terrestrial Water Balance in the Arctic
2.5 Influence of the Arctic on global climate
2.6 Arctic climate variability in the twentieth century
2.7 Arctic climate variability prior to 100 years BP
2.8 Summary and key findings of ACIA on Arctic Climate - Past and Present
References
Citation
Committee, I. (2012). Influence of the Arctic on global climate. Retrieved from http://editors.eol.org/eoearth/wiki/Influence_of_the_Arctic_on_global_climate- ↑ Broecker,W.S., G. Bond and M.A. Klas, 1990. A salt oscillator in the glacial Atlantic? 1: the concept. Paleoceanography, 5:469–477.
- ↑ Manabe, S., R.J. Stouffer, M.J. Spelman and K. Bryan, 1991.Transient response of a coupled ocean-atmosphere model to gradual changes of atmospheric CO2. Part I. Annual mean response. Journal of Climate, 4:785–818;Rind, D., R. Healy, C. Parkinson and D. Martinson, 1995.The role of sea ice in 2xCO2 climate model sensitivity. Part I:The total influence of sea ice thickness and extent. Journal of Climate, 8:449–463.
- ↑ Aagaard, K. and P. Greisman, 1975.Toward new mass and heat budgets for the Arctic Ocean. Journal of Geophysical Research, 80:3821–3827;Nikiforov,Ye.G. and A.O. Shpaikher, 1980. On the regular features of formation of large-scale oscillations of the hydrological regime of the Arctic Ocean. Gidrometeoizdat, St. Petersburg, 269pp. (In Russian)
- ↑ Broecker,W.S., 1997.Thermohaline circulation, the Achilles heel of our climate system:Will man-made CO2 upset the current balance? Science, 278:1582–1588;Broecker, W.S., 2000.Was a change in thermohaline circulation responsible for the Little Ice Age? Proceedings of the National Academy of Sciences, 97:1339–1342.
- ↑ Swift, J.H. and K. Aagaard, 1981. Seasonal transitions and water mass formation in the Iceland and Greenland seas. Deep-Sea Research A, 28:1107–1129.
- ↑ Visbeck, M.J., J. Fischer and F. Schott, 1995. Preconditioning the Greenland Sea for deep convection. Ice formation and ice drift. Journal of Geophysical Research, 100(C9):18489–18502..
- ↑ Alekseev, G.V., O.M. Johannessen, A.A. Korablev,V.V. Ivanov and D.V. Kovalevsky, 2001. Interannual variability in water masses in the Greenland Sea and adjacent areas. Polar Research, 20(2):201–208.
- ↑ Hansen, B.,W.R.Turrell and S. Østerhus, 2001. Decreasing overflow from the Nordic Seas into the Atlantic Ocean through the Faroe Bank Channel since 1950. Nature, 411:927–930.
- ↑ Dickson, R.R., I.Yashayaev, J. Meincke, B.Turrell, S. Dye and J. Holfort, 2002. Rapid freshening of the deep North Atlantic Ocean over the past four decades. Nature, 416:832–837.
- ↑ Aagaard, K. and E.C. Carmack, 1989.The role of sea ice and other fresh water in the Arctic circulation. Journal of Geophysical Research, 94(C10):14485–14498.
- ↑ IPCC, 2001b. Climate Change 2001: Synthesis Report. A Contribution of Working Groups I, II, and III to the Third Assessment Report of the Intergovernmental Panel on Climate Change.Watson, R.T., and the CoreWriting Team (eds.). Cambridge University Press, 398 pp.
- ↑ Jonasson, S.E., G.R. Shaver and F.S. Chapin III, 2001. Biogeochemistry in the Arctic: patterns, processes and controls. In: E.D. Schulze, M. Heimann, S.P. Harrison, E.A. Holland, J.J. Lloyd, I.C. Prentice and D. Schimel (eds.). Global Biogeochemical Cycles in the Climate System, pp. 139–150. Academic Press.
- ↑ IPCC, 2001c. Climate Change 2001:The Scientific Basis. Contribution of Working Group I to the Third Assessment Report of the Intergovernmental Panel on Climate Change. Houghton, J.T.,Y. Ding, D.J. Griggs, M. Noguer, P.J. van der Linden, X. Dai, K. Maskell and C.A. Johnson (eds.) Cambridge University Press, 881 pp.
- ↑ (Jonasson et al., 2001, Op. cit.
- ↑ Anisimov, O.A., N.I. Shiklomanov and F.E. Nelson, 1997. Effects of global warming on permafrost and active layer thickness: results from transient general circulation models. Global and Planetary Change, 61:61–77;Fukuda, M., 1994. Methane flux from thawing Siberian permafrost (ice complexes) results from field observations. EOS,Transactions of the American Geophysical Union, 75:86.
- ↑ Kennett, J.P., K.G. Cannariato, I.L. Hendy and R.J. Behl, 2000. Carbon isotopic evidence for methane hydrate instability during Quaternary interstadials. Science, 288:128–133.