Climate Literacy Handbook: Principle 5
Previous: Principle 4 | Table of Contents (Climate Literacy Handbook: Principle 5) |Next: Principle 6
Principle 5. Our understanding of the climate system is improved through observations, theoretical studies, and modeling |
When it comes to climate, how do scientists know what they know? While studies indicate that actively publishing climate researchers virtually all agree that human activities are altering the climate system, the general public is under the impression that scientists are still debating whether or not humans are through their activities changing climate. Essential Principle 5 concerns key elements of climate studies and the "self-correcting" peer review process.
Concept 5a. The components and processes of Earth’s climate system are subject to the same physical laws as the rest of the Universe. Therefore, the behavior of the climate system can be understood and predicted through careful, systematic study. |
It is not uncommon for the topic of climate change to be intermixed with politics in general discussions. This is understandable because climate change carries weighty implications for public policy, and politicians like Al Gore have taken a very visible role in calling for greater action and understanding. Nevertheless, climate science stands apart from politics. The scientific process works the same for every type of science, whether that science is viewed by the public as politicized or noncontroversial. Scientific organizations like NASA and NOAA are increasingly making climate data available online, which is one way for citizens to gain insight into the scientific process.
Articles in Depth:
Teaching Aids and External Resources:
- Photo Essay: Climate ScientistsA set of nine photos showing climate scientists at work, from the Guardian UK.
- Interactive: How Science Works http://www.lynnecherry.com/work1.htmAn interactive flowchart illustrating the iterative process of science and its interactions with society, from the Understanding Science website of the University of California Museum of Paleontology.
- Book: How We Know What We Know About Our Changing ClimateA widely acclaimed children's book appropriate for classroom use by author Lynne Cherry and photojournalist Gary Braasch, approaching the topic of climate change from the perspective of the scientists who are studying it.
Concept 5b. Environmental observations are the foundation for understanding the climate system. From the bottom of the ocean to the surface of the Sun, instruments on weather stations, buoys, satellites, and other platforms collect climate data. To learn about past climates, scientists use natural records, such as tree rings, ice cores, and sedimentary layers. Historical observations, such as native knowledge and personal journals, also document past climate change. |
Scientists rely on accurate, standardized measurements to study change, whether those changes are in weather, climate, organisms or the solar system beyond Earth. Today there are arrays of sophisticated instruments on the ground, in and under the water, in the air and in space above the planet recording data about the planet. While there are some gaps in the data coverage, in many areas the biggest challenge is actually the storage and analysis of the terabytes of data streaming off satellites and other instruments .
Not long ago, too much data was not the problem. In the United States, the Weather Bureau, (now the National Weather Service which is part of NOAA) was founded in 1870, and the International Meteorological Organization (now the World Meteorological Organization), was established a few years later, in 1873. It took decades before there was a network of weather stations in the United States and even longer before worldwide recording and storing of data about temperature and precipitation ensued. Satellite measurements of the Earth’s climate system began in the 1970. In addition today, cooperative networks (such as the Community Collaborative Rain, Hail & Snow Network) coordinating thousands of volunteers help fill in the spatial gaps and allow scientists to better understand regional dynamics and microclimates.
Articles in Depth:
Teaching Aids and External Resources:
- Video: Polar VisionsA set of seven short, classroom-ready video segments highlighting the work of climate scientists working in polar regions. From the University of Colorado, CIRES Education and Outreach.
- Lesson: Time and Cycles: DendrochronologyIn this activity, students can use pre-marked paper strips to simulate tree-ring core samples to help them understand that data for past climate changes can be gathered from sources beyond long-term weather observations. The instructor guide contains detailed background material about dendrochronology.
- Microwave Remote Sensing: Clouds, Precipitation, and Water Vapor- UCARThis module developed for meterologists provides an introduction to polar-orbiting-satellite-based microwave remote sensing products that depict moisture and precipitation in the atmosphere. A series of case examples highlights potential weather forecasting applications for these products. Free registration required; from UCAR and the COMET program.
- The Community Collaborative Rain, Hail & Snow NetworkThis national collaborative scientist/volunteer program is operated by the Colorado State Climatologist’s office.
Concept 5c. Observations, experiments, and theory are used to construct and refine computer models that represent the climate system and make predictions about its future behavior. Results from these models lead to better understanding of the linkages between the atmosphere-ocean system and climate conditions and inspire more observations and experiments. Over time, this iterative process will result in more reliable projections of future climate conditions. |
Given how complex the climate system is and how difficult it is to predict weather, how can computer models possibly simulate the future climate? This question is frequently raised in the public debates around climate change, but much less frequently among scientists familiar with the contributions modeling has made to climate science.
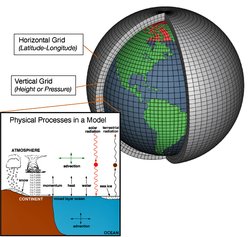
Like many technological developments, computer models had a military origin. They were first used in World War II in the development of the atomic bomb. These models rely on data and mathematical algorithms to run various scenarios. The results of different models are compared to one another; those that can replicate known data patterns with a minimum of starting data are considered to be the best estimation of the phenomenon they are studying. Once known patterns can be replicated, the models can be used to generate future predictions.
Over the past decade, the use of supercomputers have allowed climate scientists to do “hindcasts” looking at past events, even going back thousands of years, comparing the modeling results with actual paleoclimate observations. Now there are a variety of large, complex Global Climate Models (GCM) and Earth-system Models of Intermediate Complexity (EMIC) that take into account incoming and outgoing energy, and the interactions of atmosphere, oceans, land surface, ice, organisms, and human activities.
Computer models are just one type of scientific model. A scientific model is any simplified representation of a natural phenomenon based on observations. Most children build models of the solar system in school to gain a deeper understanding of the relationships between planets in the sun. Rutherford's famous model of the atom led to more and more accurate models over time, which are still being added to today. While no scientific model is perfectly accurate, they each provide some valuable insight into the working of natural systems. Like other scientific efforts, computer modeling improves over time with the combined work of many scientists and peer review.
Articles in Depth:
- Air pollution dispersion modeling
- Modeling atmospheric dispersion of pollutants
- Air pollution dispersion terminology
- Modeling of sea ice processes
Teaching Aids and External Resources:
- Wikipedia: Climate ModelsA general background article from Wikipedia.
- Book: Fundamentals Of Stack Gas DispersionA comprehensive book about the dispersion of continuous, buoyant air pollution plumes.
Concept 5d. Our understanding of climate differs in important ways from our understanding of weather. Climate scientists’ ability to predict climate patterns months, years, or decades into the future is constrained by different limitations than those faced by meteorologists in forecasting weather days to weeks into the future. |
This fundamental concept is based on “Climate Change: An Information Statement of the American Meteorological Society”:
How can climate change be projected in the future? Climate will continue to change due to natural and human causes. The most comprehensive projections of future climate rely on numerical models of the climate system, of which there are many. Climate models are complex computer codes based on measurements and on fundamental physical laws of motion, thermodynamics, and radiative transfer. These are expressed in mathematical equations representing changes of winds in the atmosphere; currents in the ocean; exchanges of heat and moisture between the atmosphere and Earth’s surface; the release of latent heat by condensation during the formation of clouds and raindrops; and the absorption of sunshine and emission of infrared radiation.
Climate models were developed from weather forecast models through coupling with models of the ocean, land surface and vegetation, cryosphere, etc., so as to represent the complexity of the climate system. Changes in the means and extremes of temperature and precipitation in response to increasing greenhouse gases can be projected over decades to centuries even though the timing of individual weather events cannot be projected. Unlike daily weather forecasts, there is limited historical basis of experience on which to judge the accuracy of climate projections. Confidence must be assessed by other methods. These include inferences from prehistoric paleoclimate evidence, and careful processstudy observations of the causal chain between energy flow changes and climate pattern responses. A useful demonstration of the validity of current climate models is their ability to reproduce the global mean temperature changes of the 20th century when (and only when) they include all known natural and human-induced climate forcings.
Weather predictions beyond a few days are nowadays based on ensembles of simulations that estimate the range of probable outcomes. The same ensemble concept is used for projections of climate change, where uncertainty arises from the limitations of models and from the emission scenarios used to represent the effects of human activity. Model limitations include uncertainties in the way in which processes that operate at scales smaller than the resolved scale of the model are represented, as well as those that arise from components of the Earth system not currently included in models. Among the most important uncertainties are changes in clouds, which can either cool or warm the climate. Recent satellite evidence rules out the possibility that cloud changes could offset most greenhouse warming and suggests that they might even add to it. The emission scenarios used to drive the climate model projections are uncertain since they depend on socioeconomic responses to climate change; these uncertainties have been factored into future assessments.
Articles in Depth:
Teaching Aids and External Resources:
- Modeling the Future of Climate ChangeA short article, written for teachers, about the application of climate models to climate change. From UCAR.
- Interactive: Model Fundamentals http://www.windows.ucar.edu/tour/link=/earth/climate/cli_models.html&portal=vocalsThis module developed for meterologists describes how weather models are designed using Numerical Weather Prediction (NWP). From UCAR.
Concept 5e. Scientists have conducted extensive research on the fundamental characteristics of the climate system and their understanding will continue to improve. Current climate change projections are reliable enough to help humans evaluate potential decisions and actions in response to climate change. |
By the year 2100, will the mean surface temperature for the planet increase a few degrees or more than a few? Will sea level rise less than a meter or much more than a meter? The models don’t all agree and, in hindsight, they tend to smooth out variability. Reality can be more variable than model runs are. For example, models have projected a steady loss of sea ice in the Arctic which has generally been borne out, but which failed to predict an abrupt loss of ice in the summer of 2007. Climate projections are considered by most climate scientists to be increasingly accurate, as they are continually fine-tuned and tap ever-increased computing power. They can help humans make decisions about the future, even if they cannot be expected to be perfect.
Articles in Depth:
Teaching Aids and External Resources:
- Models Underestimate Loss of Arctic Sea IceAn article about unexpected sea ice losses observed in the summer of 2007, from the National Snow and Ice Data Center (NSIDC).
Previous: Principle 4 | [[Table of Contents (Climate Literacy Handbook: Principle 5)]2] |Next: Principle 6