Arctic climate variability in the twentieth century
Topics: |
This is Section 2.6 of the Arctic Climate Impact Assessment Lead Author: Gordon McBean. Contributing Authors: Genrikh Alekseev, Deliang Chen, Eirik Førland, John Fyfe, Pavel Y. Groisman, Roger King, Humfrey Melling, Russell Vose, Paul H.Whitfield
Contents
Observing systems and data sources (2.6.1)
All arctic countries maintain programs of synoptic observations to support their economic activity and the sustainability of communities in the Arctic. Due to the harsh environment and the sparseness of the observation network, the need for meteorological observations is often a major (or even the only) reason for the existence of many arctic settlements. Systematic in situ arctic meteorological observations started in the late 18th century in the Atlantic sector[1]. In Fennoscandia, the oldest systematic climatic observations north of 65º N were made in Tornio, Finland, between 1737 and 1749, and regular weather stations were established around 1850. At Svalbard, the first permanent weather station was established in 1911.The first station in the Russian north was established at Arkhangelsk in 1813. Most of the meteorological network in central and northern Alaska was established in the 1920s, with the first station, Kotzebue, opening in 1897.The first meteorological observations in southern Alaska (Sitka at 57º N) were made in 1828. In northern Canada, systematic meteorological observations started in the 1940s.
Meteorological observations in the Arctic Ocean began with the first research voyage of Fridtjof Nansen onboard Fram (1894–1896). Additional observations were made during the 1920s and 1930s by ships trapped in the pack ice. A new phase of Arctic Ocean observations began in the mid-1930s with the establishment of North Pole ice stations[2].
Economic issues led to a significant reduction in the existing meteorological network in northern Russia and Canada in the 1990s. Thus, during the past decade, the number of arctic meteorological stations has noticeably decreased, and the number of the stations conducting atmospheric measurements using balloons has decreased sharply.
The national meteorological services of the Nordic countries, Canada, Russia, and the United States maintain extensive archives of in situ observations from their national networks. The station density in these networks varies substantially, from 2 per 1,000 km2 in Fennoscandia to 1 per 100,000 km2 in Canada north of 60º N, northern Alaska, and (since the 1990s) in northern regions of Siberia.
In seeking to assemble a high-quality record, different levels of quality assurance, data infilling, and homogenization adjustments are required.The Global Historical Climatology Network (GHCN) dataset includes selected quality controlled long-term stations suitable for climate change studies, while the Global Daily Climatology Network dataset goes through a more limited screening. The Integrated Surface Hourly Dataset incorporates all synoptic observations distributed through the Global Telecommunication System during the past 30 years. All rawindsonde observations in the Arctic (300 stations north of 50º N and 135 stations north of 60º N) are currently collected in the Comprehensive Aerological Reference Data Set.
The sea-ice boundaries in the Atlantic sector of the Arctic Ocean have been documented since the beginning of the 20th century. Since the late 1950s, sea-ice observations have been conducted throughout the year. The development of shipping along the coast of Siberia in the mid-1920s led to sea-ice monitoring in Siberian Arctic waters. By the late 1930s, aviation had become the main observation tool; since the 1970s, satellite remote sensing has been used.The notes of seamen and the logs of fishing, whaling, and sealer vessels operating in arctic waters serve as an important source of information about changes in the state of arctic sea ice throughout the 19th and 20th centuries[3]. A significant amount of historical data on sea ice near the shores of Iceland was preserved and generalized in many studies[4]. Information on sea-ice thickness is scarce; observations of ice draft using upward-looking sonar from submarines and stationary systems are the primary source of this information (see Section 2.3.4 (Arctic climate variability in the twentieth century)).
Sea-ice data are concentrated at two World Data Centers. Datasets of satellite observations of sea-ice and snow-cover extent[5], snow water equivalent from the Special Sensor Microwave Imager[6], cloudiness[7], and the radiation budget[8] are available from the National Aeronautics and Space Administration (Goddard Institute for Space Studies, Langley Atmospheric Sciences Data Center) and the National Oceanic and Atmospheric Administration (National Climatic Data Center, National Snow and Ice Data Center – NSIDC). A suite of arctic-related datasets is available from the NSIDC (http://nsidc.org/index.html).
Oceanographic measurements in the central Arctic were initiated in 1894 by Nansen[9] (1906).They were restarted in the 1930s, interrupted during the Second World War, and resumed in the late 1940s with the help of aviation and drifting stations. Since 1987, icebreakers with conductivity, temperature, and depth sondes have been used to make observations.
The hydrologic network in the Arctic is probably the weakest of the arctic observation networks, which makes information about the arctic water budget quite uncertain[10]. A circumpolar river discharge dataset is available online[11].
Atmospheric changes (2.6.2)
Land-surface air temperature (2.6.2.1)
Although several analyses[12] have examined large-scale temperature variations in the Arctic, no study has evaluated the spatial and temporal variations in temperature over all land areas in the zone from 60º to 90º N for the entire 20th century. Consequently, temperature trends are illustrated for this latitude band using the Climatic Research Unit (CRU) database[13] and the GHCN database[14]. Both databases were used in the IPCC Third Assessment Report[15] to summarize the patterns of temperature change over global land areas since the late 19th century.While the impact of urbanization on large-scale temperature trends in the Arctic has not been assessed, the results of Jones et al.[16], Easterling et al.[17], Peterson et al.[18], and Peterson[19] indicate that urbanization effects at the global, hemispheric, and even regional scale are small (<0.05 ºC over the period 1900 to 1990).
Figure 2.6 depicts annual land-surface air temperature variations in the Arctic (north of 60º N) from 1900 to 2003 using the GHCN dataset.The CRU time series is virtually identical to the GHCN series, and both document a statistically significant warming trend of 0.09 ºC/decade during the period shown (Table 2.1). The arctic trend is greater than the overall Northern Hemisphere trend of 0.06 ºC/decade over the same period[20]. In general, temperature increased from 1900 to the mid-1940s, then decreased until about the mid-1960s, and increased again thereafter. The general features of the arctic time series are similar to those of the global time series, but decadal trends and interannual variability are greater in the Arctic. Comparisons of the arctic and global time series are discussed later in this section.
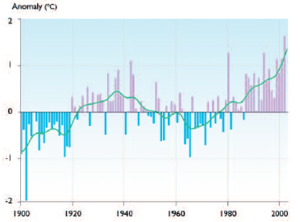
1900–2003 |
1900–1945 |
1946–1965 |
1966–2003 | |
GHCN dataset |
0.09a |
0.29a |
-0.14 |
0.40a |
CRU dataset |
0.09a |
0.31a |
-0.20 |
0.40a |
Figure 2.7 illustrates the patterns of land-surface air temperature change in the Arctic between 1900 and 2003 and for three periods therein.Trends were calculated from annually averaged gridded anomalies using the method of Peterson et al. (1999) with the requirement that annual anomalies include a minimum of ten months of data. For the period 1900 to 2003, trends were calculated only for those 5º x 5º grid boxes with annual anomalies in at least 70 of the 104 years.The minimum number of years required for the shorter time periods (1900– 1945, 1946–1965, and 1966–2003) was 31, 14, and 26, respectively.The three periods selected for this figure correspond approximately to the warming–cooling– warming trends seen in Fig. 2.6.The spatial coverage of the region north of 60º N is quite varied. During the first period (1900–1945), there are only three stations meeting the requirement of 31 years of data in Region 4 and only four in Region 3 (see section 18.3 for map and description of the ACIA regions).The highest density of stations is found in Region 1.The coverage for the second and third periods is more uniform.
Although it is difficult to assess trends in some areas, air temperature appears to have increased throughout the Arctic during most of the 20th century.The only period characterized by widespread cooling was 1946 to 1965, and even then large areas (e.g., southern Canada and southern Eurasia) experienced significant increases in temperature.Temperatures in virtually all parts of the Arctic increased between 1966 and 2003, with trends exceeding 1 to 2 ºC/decade in northern Eurasia and northwestern North America.The average trend between 1966 and 2003 over the Arctic was 0.4 ºC/decade, approximately four times greater than the average for the century.While most pronounced in winter and spring, all seasons experienced an increase in temperature over the past several decades (Fig. 2.8).
The trends shown in Fig. 2.8 were calculated from seasonally-averaged gridded anomalies using the method of Peterson et al.[22] with the requirement that the calculation of seasonal anomalies should include all three months.Trends were calculated only for those 5º x 5º grid boxes containing seasonal anomalies in at least 26 of the 38 years.The updated analysis by Chapman and Walsh[23] showed generally similar patterns of change over the period 1954 to 2003, but with areas of cooling around southern Greenland.
The instrumental record of land-surface air temperature is qualitatively consistent with other climate records in the Arctic[24]. For instance, temperatures in the marine Arctic (as measured by coastal land stations, drifting ice stations, and Russian North Pole stations) increased at the rate of 0.05 ºC/decade during the 20th century[25]. As with the land-only record, the increases were greatest in winter and spring, and there were two relative maxima during the century (the late 1930s and the 1990s). For periods since 1950, Polyakov et al.[26] found the rate of temperature increase in the marine Arctic to be similar to that noted for the GHCN dataset. Because of the scarcity of data prior to 1945, it is very difficult to say whether the Arctic as a whole was as warm in the 1930s and 1940s as it was during the 1990s. In the Polyakov et al.[27] analysis, only coastal stations were chosen and most of the stations contributing to the high average temperatures in the 1930s were in Scandinavia. Interior stations, especially those between 60º N (the southern limit for the analysis in this section) and 62º N (the southern limit for the Polyakov et al.[28] study), have warmed more than coastal stations over the past few decades. As discussed in section 2.6.3, arctic sea-ice extent contracted from 1918 to 1938 and then expanded between 1938 and 1968[29]. The expansion after 1938 implies that the Arctic was cooling during that period.
Polyakov et al.[30] presented the temperature trends (ºC/yr) for their dataset (as described above) and for the Jones et al.[31] Northern Hemisphere dataset. For comparison purposes, the temperature trends (ºC/yr) for the land-surface temperatures (GHCN dataset) were computed for the latitude bands 60º to 90º N and 0º to 60º N (Fig. 2.9).The trend shown for any given year before present is the average trend from that year through 2003; for example, the value corresponding to 60 years before present is the average trend for the period 1944 to 2003. In both latitudinal bands, the trend over any period from 120 years ago to the present is positive (i.e., the Arctic is warming). Although the trends for both bands have been increasing over the past 60 years, the trend in the 60º to 90º N band is larger. The rate of temperature increase in the Arctic (as defined here) exceeds that of lower latitudes. Due to natural variability and sparse data in the Arctic, the arctic trend shows more variability and the confidence limits are wider. Over the past 40 years, the arctic warming trend was about 0.04 ºC/yr (0.4 ºC/decade) compared to a trend of 0.025 ºC/yr for the lower latitudes.
Likewise, surface temperatures derived from satellite thermal infrared measurements, which provide circumpolar coverage from 1981 to 2001, exhibited statistically significant warming trends in all areas between 60º to 90º N except Greenland[32]. The warming trends were 0.33 ºC/decade over the sea ice, 0.50 ºC/ decade over Eurasia, and 1.06 ºC/decade over North America. In addition, the recent reduction in sea-ice thickness[33], the retreat of sea-ice cover[34], and the decline in perennial sea-ice cover[35] are consistent with large-scale warming in the Arctic. In view of this evidence, it is very probable that the Arctic has warmed over the past few decades.
Global climate model simulations generally indicate that increasing atmospheric GHG concentrations will result in greater temperature increases in the Arctic than in other parts of the world. As stated by the IPCC[36], model experiments show “a maximum warming in the high latitudes of the Northern Hemisphere”. In reference to warming at the global scale, the IPCC[37] also concluded, “There is new and stronger evidence that most of the warming observed over the past 50 years is attributable to human activities”.
The question is whether there is definitive evidence of an anthropogenic signal in the Arctic.This would require a direct attribution study of the Arctic, which has not yet been done.There are studies showing that an anthropogenic warming signal has been detected at the regional scale. For example, Karoly et al.[38] concluded that temperature variations in North America during the second half of the 20th century were probably not due to natural variability alone. Zwiers and Zhang[39] were able to detect the combined effect of changes in GHGs and sulfate aerosols over both Eurasia and North America for this period, as did Stott et al.[40] for northern Asia (50º–70º N) and northern North America (50º–85º N). In any regional attribution study, the importance of variability must be recognized. In climate model simulations, the arctic signal resulting from GHG-induced warming is large but the variability (noise) is also large. Hence, the signal-to-noise ratio may be lower in the Arctic than at lower latitudes. In the Arctic, data scarcity is another important issue.
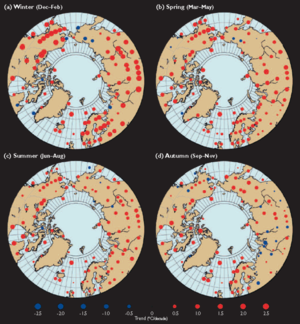
A related question is whether the warming in the Arctic is enhanced relative to that of the globe (i.e., polar amplification). For example, Polyakov et al.[42] concluded that observed trends in the Arctic over the entire 20th century did not show polar amplification. A number of studies[43] suggested that much of the recent warming resulted from changes in atmospheric circulation; specifically, during the past two decades when the AO was in a phase that brought relatively warm air to the same areas that experienced the greatest increases in temperature (i.e., northern Eurasia and northwestern North America). Serreze et al.[44], however, noted that the changes in circulation were “not inconsistent” with anthropogenic forcing.
It is clear that trends in temperature records, as evidence of polar amplification, depend on the timescale chosen. Over the past 100 years, it is possible that there has been polar amplification but the evidence does not allow a firm conclusion. As noted previously, the analysis presented here of land stations north of 60º N does show more warming over the past 100 years (0.09 ºC/decade for the Arctic compared to 0.06 ºC/decade for the globe). However, an analysis of coastal stations north of 62º N[45] found arctic warming of 0.05 ºC/decade over the past 100 years. Johannessen et al.[46] found, with a more extensive dataset, that the “early warming trend in the Arctic was nearly as large as the warming trend for the last 20 years” but “spatial comparison of these periods reveals key differences in their patterns”.The pattern of temperature increases over the past few decades is different and more extensive than the pattern of temperature increases during the 1930s and 1940s, when there was weak (compared to the present) lower-latitude warming.
In conclusion, for the past 20 to 40 years there have been marked temperature increases in the Arctic.The rates of increase have been large, and greater than the global average. Two modeling studies have shown the importance of anthropogenic forcing over the past half century for modeling the arctic climate. Johannessen et al.[47]used a coupled atmosphere–ocean general circulation model (AOGCM) to study the past 100 years and noted, “It is suggested strongly that whereas the earlier warming was natural internal climate-system variability, the recent SAT (surface air temperature) changes are a response to anthropogenic forcing”. Goosse and Renssen[48] simulated the past 1,000 years of arctic climate with a coarser resolution AOGCM and were able to replicate the cooling and warming until the mid-20th century.Without anthropogenic forcing, the model simulates cooling after a temperature maximum in the 1950s.There is still need for further study before it can be firmly concluded that the increase in arctic temperatures over the past century and/or past few decades is due to anthropogenic forcing.
Precipitation (2.6.2.2)
Precipitation assessments in the Arctic are limited by serious problems with the measurement of rainfall and snowfall in cold environments[49]. Methods for correcting measured precipitation depend upon observing practices, which differ between countries; in addition, metadata (information about the data) needed to perform corrections are often inadequate. The IPCC[50] concluded, “It is very likely that precipitation has increased by 0.5 to 1% per decade in the 20th century over most mid- and high latitudes of the Northern Hemisphere continents”.
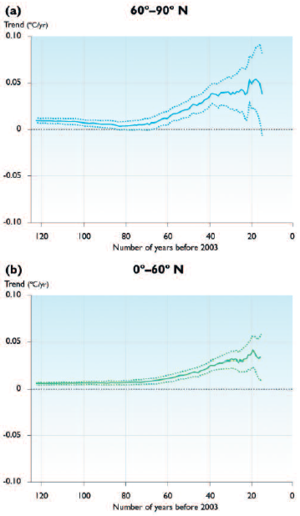
An updated assessment of precipitation changes north of 60º N is presented in Figs. 2.10 and 2.11. These figures provide estimates of linear trends in annual and seasonal precipitation based on available data in the GHCN database[52].Trends were calculated from annually-averaged gridded anomalies (baseline 1961–1990) using the method of Peterson et al.[53] with the requirement that annual anomalies include a minimum of ten months of data (Fig. 2.10) and that seasonal anomalies must contain all three months (Fig. 2.11). In Fig. 2.10, for the period 1900 to 2003 trends were calculated only for those 5º x 5º grid boxes containing annual anomalies in at least 70 of the 104 years; the minimum number of years required for the shorter time periods (1900–1945, 1946–1965, and 1966–2003) was 31, 14, and 26 respectively. In Fig. 2.11, trends were calculated only for those 5º x 5º grid boxes containing seasonal anomalies in at least 26 of the 38 years.
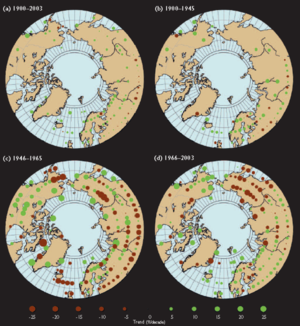
For the entire period from 1900 to 2003,Table 2.2 indicates a significant positive trend of 1.4% per decade. New et al.[55] used uncorrected records and found that terrestrial precipitation averaged over the 60º to 80º N band exhibited an increase of 0.8% per decade over the period from 1900 to 1998. In general, the greatest increases were observed in autumn and winter[56]. Fig. 2.10 shows that most high-latitude regions have experienced an increase in annual precipitation. During the arctic warming in the first half of the 20th century (1900– 1945), precipitation increased by about 2% per decade, with significant positive trends in Alaska and the Nordic region. During the two decades of arctic cooling (1946–1965), the high-latitude precipitation increase was roughly 1% per decade. However, there were large regional contrasts with strongly decreasing values in western Alaska, the North Atlantic region, and parts of Russia. Since 1966, annual precipitation has increased at about the same rate as during the first half of the 20th century. In eastern Russia, annual precipitation decreased, mostly because of a substantial decrease during winter (Fig. 2.11). Average Alaskan precipitation has increased in all seasons.
These trends are in general agreement with the results of a number of regional studies. For example, there have been positive trends in annual precipitation (up to a 20% increase) during the past 40 years over Alaska[57], Canada north of 55º N[58], and the Russian permafrost-free zone[59]. Siberian precipitation exhibited little change during the second half of the 20th century[60]. Hanssen-Bauer et al.[61] documented that annual precipitation in northern Norway increased by 15% during the 20th century. Førland et al.[62] and Hanssen-Bauer and Førland[63] found precipitation increases of about 25% over the past 80 years in Svalbard. Lee et al.[64] described details of specific periods and changes in annual precipitation, which generally increased between 1880 and 1993 in the Arctic.
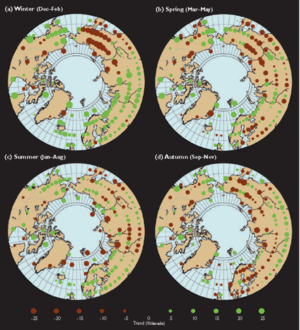
Overall, it is probable that there was an increase in arctic precipitation over the past century.
A few studies suggest that the fraction of annual precipitation falling as snow has diminished, which is consistent with widespread temperature increases in the Arctic. For the period 1950 to 2001, Groisman et al.[66] found an increase of 1.2% per decade in annual rainfall north of 50º N, an increase that was partially due to an additional fraction of liquid precipitation during spring and autumn. In addition, Førland and Hanssen-Bauer[67] found that the fraction of solid precipitation diminished at all stations in the Norwegian Arctic between 1975 and 2001. If the fraction of precipitation falling in solid form has decreased, it is possible that the arctic trend in total precipitation may be overestimated because gauge under-catch is less problematic during liquid precipitation events[68].
Reporting changes in precipitation frequency in cold environments is difficult because many events result in small precipitation totals and many gauges have accuracy problems during light precipitation events.To avoid uncertainties in the measurement of small amounts of precipitation, Groisman et al.[69] assessed the climatology and trends in the annual number of days with precipitation >0.5 mm for the period 1950 to 2001. They found no significant change in the number of wet days over North America and northern Europe (including western Russia) but a significant decrease in wet days over eastern Russia.The latter was first reported by Sun et al.[70] for eastern Russia south of 60º N and is remarkable because it is accompanied by an increase in heavy precipitation in the same region[71].
The IPCC[72] concluded that it was likely that there had been a widespread increase in heavy and extreme precipitation events in regions where total precipitation had increased (e.g., in the high latitudes of the Northern Hemisphere). Subsequent to the study of Groisman et al.[73], which reported that heavy precipitation events appear to have increased at high latitudes, a series of analyses demonstrated that changes in heavy precipitation over Canada are spatially diverse[74]. Mekis and Hogg[75] noted that the magnitude of heavy precipitation events making up the top 10% of such events increased in northern Canada but decreased in the south during the 20th-century period of record. Zhang X. et al.[76] reported a marked decadal increase in heavy snowfall events in northern Canada. Stone et al.[77] showed that during the second half of the 20th century, heavy and intermediate daily precipitation events became significantly more frequent in over one-third of all districts of Canada, indicating a shift to more intense precipitation. Groisman et al.[78] reported an increase in the frequency of “very heavy” (upper 0.3%) rain events over the European part of Russia during the past 65 years. Comparing two multidecadal periods in the second half of the 20th century, Frich et al.[79] reported an increase in most indicators of heavy precipitation events over western Russia, eastern Canada, and northern Europe, while Siberia showed a decrease in the frequency and/or duration of heavy precipitation events.
Sea-level pressure (2.6.2.3)
Sea-level pressure data from the Arctic Buoy Program (1979–1994) show decreasing pressures in the central arctic that are greater than decreases in sea-level pressure found anywhere else in the Northern Hemisphere[80].These decreases are greatest and statistically significant in autumn and winter, and are compensated by pressure increases over the subpolar oceans. It is very probable that sea-level pressure has been dropping over the Arctic during the past few decades.A useful review of other evidence for these and related changes, and their linkages to the AO, was undertaken by Moritz et al.[81]. A similar pattern of sealevel pressure change is simulated by some climate models when forced with observed variations in GHGs and tropospheric sulfate aerosols[82]. However, for unknown reasons, the magnitude of the anthropogenically forced pressure change in climate models is generally much less than the observed pressure change. Longer-term (>100 years) observations, based in part on recently released Russian meteorological observations from coastal stations, show that the decrease in central arctic sea-level pressure during recent decades is part of a longer-term (50 to 80 year) oscillation that peaked in the late 1800s and again in the 1950s and 1960s[83].This suggests that the decrease in central arctic sea-level pressure during recent decades may be a result of the combined effects of natural and anthropogenic forcing.
1900–2003 |
1900–1945 |
1946–1965 |
1966–2003 | |
GHCN dataset |
1.4a |
2.3a |
1.3 |
2.2 |
Other variables (2.6.2.4)
Groisman et al.[85] assessed a set of atmospheric variables derived from daily temperatures and precipitation that have applications in agriculture, architecture, power generation, and human health management. This section provides examples of these derived variables, including a temperature derivative (frequency of thaws) and a temperature and precipitation derivative (rain-on-snow-events). A day with thaw (i.e., snowmelt) can be defined as a day with snow on the ground when the daily mean temperature is above -2 ºC[86]. During these days, snow deteriorates, changes its physical properties, and (eventually) disappears. In win- ter and early spring at high latitudes, thaws negatively affect transportation, winter crops, and the natural environment including vegetation and wild animals. In late spring, intensification of thaw conditions leads to earlier snow retreat and spring onset[87]. Gradual snowmelt during the cold season affects seasonal runoff in northern watersheds, reducing the peak flow of snowmelt origin and increasing the mid-winter low flow[88]. Figures 2.12 and 2.13 show the climatology of thaws in North America and Russia and quantitative estimates of change during the past 50 years.
The time series shown in Fig. 2.13 demonstrate statistically significant increasing trends for winter and autumn of 1.5 to 2 days per 50 years.This change constitutes a 20% (winter) to 40% (autumn) increase in the thaw frequency during the second half of the 20th century. Rain falling on snow causes a faster snowmelt and, when the rainfall is intense, may lead to flash floods. Along the western coast of North America, rain-on-snow events are the major cause of severe flash floods[89]. Groisman et al.[90] found a significant increase in the frequency of winter rain-on-snow events in western Russia (50% over the 50 years from 1950 to 2000) and a significant reduction (of similar magnitude) in western Canada. During spring, there has been a significant increase in rain-on-snow events in Russia (mostly in western Russia), but there has also been a significant decrease in the frequency of these events in western Canada.The decrease in western Canada is mostly due to reduced snow-cover extent.
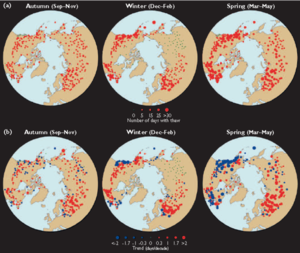
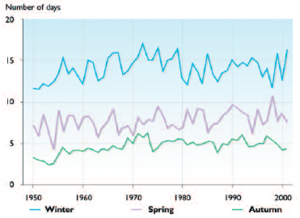
Marine Arctic (2.6.3)
The 20th-century record of sea-ice extent reveals phases of expansion between 1900 and 1918 and between 1938 and 1968, and of contraction between 1918 and 1938 and from 1968 to the present[93]. The IPCC[94] concluded, “Northern Hemisphere spring and summer sea-ice extent has decreased by about 10 to 15% since the 1950s. It is likely that there has been about a 40% decline in Arctic sea-ice thickness during late summer to early autumn in recent decades and a considerably slower decline in winter sea-ice thickness”. Since 1978, the average rate of decrease has been 2.9±0.4% per decade (Fig. 2.14;[95]). The extent of perennial sea ice has decreased at more than twice this rate[96].The contraction has been greatest in August and September, while in January and February there has been a small expansion[97]. Recent trends vary considerably by region. In eastern Canadian waters, ice cover has actually increased [98]. Interdecadal variations are large and dominated by anomalies of opposite sign in the Eurasian and American sectors[99].These variations are associated with anomalies in atmospheric pressure and temperature that closely resemble those associated with the AO (section 2.2).
The thickness of sea ice has decreased by 42% since the mid-1970s within a band across the central Arctic Ocean between the Chukchi Sea and Fram Strait[100]. In the 1990s, the average thickness in this zone at the end of summer was only 1.8 m. However, the extent to which this change is a result of greater melt and reduced growth (namely a warming climate) remains to be resolved. Thick sea ice observed before the 1990s may simply have been driven out of the band of observation by wind changes in the 1990s associated with the AO[101].The impacts of the concurrent inflow of warmer Atlantic water and disappearing cold halocline (see below) are also unclear[102]. There are no systematic trends over the past 50 years in the thickness of coastal land-fast ice in either northern Canada or Siberia[103]. Interannual variability in the thickness of coastal land-fast ice is dominated by changes in winter snow accumulation, not air temperature.
Sea-ice drift in the central Arctic Ocean has been monitored since 1979 under the direction of the International Arctic Buoy Program.The pattern of sea-ice drift changed in the late 1980s coincident with the change in the pattern of surface winds caused by the AO. In the first decade of observations, the transpolar drift of sea ice moved directly from the Laptev Sea to Fram Strait.The Beaufort Gyre extended from the Canadian Archipelago to Wrangel Island. During the 1990s, the source of the transpolar drift moved eastward into the East Siberian Sea and the Beaufort Gyre shrank eastward into the Beaufort Sea[104].The change in circulation moved the thickest sea ice toward the Canadian Archipelago and may have facilitated increased export of perennial sea ice across the north of Greenland and out through Fram Strait[105]. Section 6.3 provides additional information regarding sea ice.
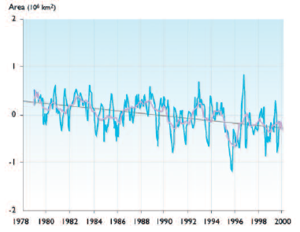
There are few systematic long-term oceanographic observations in the marine Arctic, except perhaps in the European sector. Russian airborne surveys are the primary source of information for the central Arctic prior to 1990[106]. Because most records are of short duration, confidence in discriminating between trends and variability in the marine Arctic is low. Most evidence of change in ice-covered waters is recent, emerging from observations made in the 1990s. Temperature and salinity at intermediate depths in the arctic basins were stable between 1950 and 1990. In the early 1990s, the temperature of the Fram Strait Branch north of the Barents Sea increased by 2 ºC.The temperature increase has since spread around the perimeter of the basin, reaching the Beaufort Sea north of Alaska in 2001[107]. The spatial pattern has remained consistent with that of past decades, with the highest temperatures in the eastern sector[108]. However, the ocean front that separates waters of Atlantic and Pacific origin in the Arctic moved 600 km eastward along the Siberian slope in the early 1990s, shifting its alignment from the Lomonosov Ridge to the Alpha-Mendeleyev Ridge[109]. This shift reflects a 20% increase in the volume of Atlantic water within the Arctic Ocean.The cold halocline separating sea ice from warm Atlantic water in the Eurasian Basin virtually disappeared in the 1990s[110]. In general, the pattern of spreading and the greater thickness of the Atlantic layer are consistent with increases in the rate of inflow and temperature of Atlantic water entering the Arctic via Fram Strait[111].
The magnitude and temperature of inflow to the Arctic via the Barents Sea is highly variable, in response to wind changes associated with the AO[112]. Both the inflow and its temperature have increased since 1970, mirroring trends observed during the arctic warming of 1910 to 1930[113]. The properties of the surface layer of the Arctic Ocean vary greatly by season and from year to year. Russian data reveal an average 1.3 m decrease in surface (upper 50 m) “freshwater” storage in the Arctic Ocean in winter between 1974 and 1977[114]. Surface-layer variability is greatest in the area of seasonal sea ice because varying ice conditions strongly modulate the temperature and salinity of the upper ocean[115]. Possible temporal trends in most peripheral seas of the marine Arctic remain obscured by high variability.
In the decade following 1963 there was an obvious freshening of the upper waters of the North Atlantic (the Great Salinity Anomaly[116]) which is thought to have originated in the Arctic Ocean[117]. Unusually warm summers from 1957 to 1962 in Baffin Bay and the Canadian Archipelago may have promoted melting of land ice and an enhanced freshwater outflow[118]. The Great Salinity Anomaly, first evident north of Iceland[119], peaked in the North Atlantic in 1969[120], at the same time that unusually fresh and cold water was observed on the other side of Greenland[121].The simultaneous development of freshening in both areas indicates paths for “freshwater” transport via both Fram Strait and the Canadian Archipelago.A new freshwater pulse may appear in the North Atlantic in response to lengthening of the arctic summer melt period in the 1990s[122]. For further discussion of change in the Atlantic sector, refer to section 9.2.4.2.
In the central Greenland Sea, change in the temperature of water at the 2000 m depth is an indicator of varying deep-water formation[123].The observation of a large temperature increase (0.25 ºC) in the central Greenland Sea in the 1990s is consistent with the weakening of the thermohaline circulation implied by decreasing deep-water flow toward the Arctic through the Faroe-Shetland Strait[124].
Terrestrial system (2.6.4)
About 24.5% of the exposed land surface in the Northern Hemisphere is underlain by permafrost[125], and any increase in temperature is very likely to cause this area to shrink, in turn affecting arctic groundwater supply. Section 6.6.1.2 provides a more detailed description of recent changes in permafrost. Permafrost is very sensitive to climate change, and is dependent upon ambient temperatures for its existence and distinctive properties[126]. Brown J. et al.[127] indicated that only the thin active (seasonally thawed) layer of permafrost responds immediately to temperature changes, making it both an indicator and a product of climate change, as its depth is dependent on temperature.Vourlitis and Oechel[128] reported an annual increase of 10 to 22 cm in the depth of the active layer between June and August in some permafrost areas. Climate change is very likely to accelerate this depth increase, due to both higher temperatures and increased evapotranspiration, which makes the soil drier throughout the active layer and causes a descent of the permafrost table[129].
Along the land-sea margin of the Arctic, over the past few decades there has been an increase in the magnitude of oscillations of the permafrost bottom (deepest extent of permafrost), and a greater variability in thickness during the warmer part of longer-term cycles, indicating an overall degradation of coastal permafrost[130]. Along a north– south transect in Alaska, permafrost temperatures at 15 to 20 m depths have increased between 0.6 and 1.5 ºC over the past 20 years, mean annual ground surface temperatures have increased by 2.5 ºC since the 1960s, and discontinuous permafrost has begun thawing downward at a rate of about 0.1 m/yr at some locations[131].The effects of increased permafrost temperatures on groundwater could possibly include the development of large, unfrozen near-surface aquifers with groundwater flow throughout the year; more active recharge and discharge of aquifers and increased groundwater flow would affect the base flow volumes and chemistry of many rivers.
Romanovsky and Osterkamp[132] took a series of precise permafrost temperature measurements from four observation sites in Alaska, and found that unfrozen water has the greatest effect on the ground thermal regime immediately after freeze-up and during the cooling of the active layer, but is less important during the warming and thawing of the active layer.
Jorgenson et al.[133] showed widespread permafrost degradation in the Tanana Valley due to increases in air and permafrost temperatures. Permafrost temperatures have increased in Alaska, and decreased in eastern Canada[134]. Increased permafrost temperatures have also been observed in Siberia[135]. Climate change is likely to drastically affect wildlife habitat, as permafrost is essential to the existence and functioning of arctic wetlands.
Chapter 2: Arctic Climate - Past and Present
2.1 Introduction (Arctic climate variability in the twentieth century)
2.2 Arctic atmosphere
2.3 Marine Arctic
2.4 Terrestrial Water Balance in the Arctic
2.5 Influence of the Arctic on global climate
2.6 Arctic climate variability in the twentieth century
2.7 Arctic climate variability prior to 100 years BP
2.8 Summary and key findings of ACIA on Arctic Climate - Past and Present
References
Citation
Committee, I. (2012). Arctic climate variability in the twentieth century. Retrieved from http://editors.eol.org/eoearth/wiki/Arctic_climate_variability_in_the_twentieth_century- ↑ Tabony, R.C., 1981. A principal component and spectral analysis of European rainfall. Journal of Climatology, 1:283–294.)
- ↑ Arctic Climatology Project, 2000. Environmental Working Group Arctic Meteorology and Climate Atlas. F. Fetterer and V. Radionov (eds.). National Snow and Ice Data Center, Boulder, Colorado. CD-ROM.
- ↑ Vinje,T., 2001. Fram Strait ice fluxes and atmospheric circulation:1950–2000. Journal of Climate, 14:3508–3517.
- ↑ Ogilvie, A.E.J. and I. Jónsdóttir, 2000. Sea ice, climate, and Icelandic fisheries in the eighteenth and nineteenth centuries. Arctic, 53(4):383–394.
- ↑ Ramsay, B.H., 1998.The interactive multi-sensor snow and ice mapping system. Hydrological Processes, 12:1537–1546.
- ↑ Armstrong, R.L. and M.J. Brodzik, 2001. Recent northern hemisphere snow extent: A comparison of data derived from visible and microwave satellite sensors. Geophysical Research Letters, 28:3673–3676;Grody, N.C. and A.N. Basist, 1996. Global identification of snowcover using SSM/I measurements. IEEE Transactions on Geoscience and Remote Sensing, 34:237–249.
- ↑ Rossow,W.B. and R.A. Schiffer, 1999.Advances in understanding clouds from ISCCP. Bulletin of the American Meteorological Society, 80:2261–2287.
- ↑ Wielicki, B.A., R.D. Cess, M.D. King, D.A. Randall and E.F. Harrison, 1995. Mission to planet Earth: role of clouds and radiation in climate. Bulletin of the American Meteorological Society, 76:2125–2153.
- ↑ Nansen, F., 1906. Northern waters: Captain Roald Amundsen’s oceanographic observations in the Arctic Seas 1901.Videnskabs-Selskabets Skrifter, I, Matematisk-Naturvidenskabelig Klasse, No. 3, 145pp
- ↑ Vörösmarty, C.J., L.D. Hinzman, B.J. Peterson, D.H. Bromwich, L.C. Hamilton, J. Morison,V.E. Romanovsky, M. Sturm and R.S.Webb, 2001.The Hydrologic Cycle and Its Role in Arctic and Global Environmental Change: A Rationale and Strategy for Synthesis Study. Arctic Research Consortium of the U.S., Fairbanks, Alaska, 84pp
- ↑ R-ArcticNET, 2003. A Regional, Electronic, Hydrographic Data Network for the Arctic Region. www.r-arcticnet.sr.unh.edu.
- ↑ e.g., Comiso, J., 2003.Warming trends in the Arctic from clear sky satellite observations. Journal of Climate, 16:3498–3510;Polyakov, I.V., R.V. Bekryaev, G.V. Alekseev, U.S. Bhatt, R.L. Colony, M.A. Johnson,A.P. Makshtas and D.Walsh, 2003b.Variability and trends of air temperature and pressure in the maritime Arctic, 1875–2000. Journal of Climate, 16:2067–2077.
- ↑ Jones, P.D. and A. Moberg, 2003. Hemispheric and large-scale surface air temperature variations: an extensive revision and an update to 2001. Journal of Climate, 16:206–223.
- ↑ (updated from Peterson and Vose, 1997)
- ↑ IPCC, 2001c. Climate Change 2001:The Scientific Basis. Contribution of Working Group I to the Third Assessment Report of the Intergovernmental Panel on Climate Change. Houghton, J.T.,Y. Ding, D.J. Griggs, M. Noguer, P.J. van der Linden, X. Dai, K. Maskell and C.A. Johnson (eds.) Cambridge University Press, 881 pp.
- ↑ Jones, P.D., P.Ya. Groisman, M. Coughlan, N. Plummer,W.C.Wang and T.R. Karl, 1990. Assessment of urbanization effects in time series of surface air temperature over land. Nature, 347:169–172.
- ↑ Easterling, D.R., B. Horton, P.D. Jones,T.C. Peterson,T.R. Karl, D.E. Parker, M.J. Salinger,V. Razuvayev, N. Plummer, P. Jamason and C.K. Folland, 1997. Maximum and minimum temperature trends for the globe. Science, 277:364–367.
- ↑ Peterson,T.C., K.P. Gallo, J. Livermore,T.W. Owen, A. Huang and D.A. McKittrick, 1999. Global rural temperature trends. Geophysical Research Letters, 26:329–332.
- ↑ Peterson,T.C., 2003. Assessment of urban versus rural in situ surface temperatures in the contiguous United States: no difference found. Journal of Climate, 16:2941–2959.
- ↑ IPCC, 2001b. Climate Change 2001: Synthesis Report. A Contribution of Working Groups I, II, and III to the Third Assessment Report of the Intergovernmental Panel on Climate Change.Watson, R.T., and the CoreWriting Team (eds.). Cambridge University Press, 398 pp.
- ↑ Updated from Peterson,T.C. and R.S.Vose, 1997. An overview of the Global Historical Climatology Network temperature database. Bulletin of the American Meteorological Society, 78:2837–2849.
- ↑ Peterson et al., (1999), Op. cit.
- ↑ Chapman,W.L. and J.E.Walsh, 2003. Observed climate change in the Arctic, updated from Chapman and Walsh, 1993. Recent variations of sea ice and air temperatures in high latitudes. Bulletin of the American Meteorological Society, 74(1):33–47. <a class="external free" href="http://arctic.atmos.uiuc.edu/" rel="nofollow" title="http://arctic.atmos.uiuc.edu/">http://arctic.atmos.uiuc.edu/</a> CLIMATESUMMARY/2003/
- ↑ Serreze, M.C., J.E.Walsh, F.S. Chapin III,T. Osterkamp, M. Dyurgerov, V. Romanovsky,W.C. Oechel, J. Morison,T. Zhang and R.G. Barry, 2000. Observational evidence of recent change in the northern high latitude environment. Climatic Change, 46:159–207.
- ↑ Polyakov et al., 2003b, Op. cit.
- ↑ Polyakov et al. 2003b Op. cit.
- ↑ Ibid.
- ↑ Ibid.
- ↑ Zakharov,V.F., 2003. Sea ice extent changes during XX century. Meteorology and Hydrology, 5:75–86 (in Russian).
- ↑ Polyakov, I.V., G.V. Alekseev, R.V. Bekryaev, U. Bhatt, R.L. Colony, M.A. Johnson,V.P. Karklin,A.P. Makshtas, D.Walsh and A.V.Yulin, 2002. Observationally based assessment of polar amplification of global warming. Geophysical Research Letters, 29(18):1878, doi:10.1029/2001GL011111.
- ↑ Jones P.D., M. New, D.E. Parker, S. Martin and I.G. Rigor, 1999. Surface air temperature and its changes over the past 150 years. Reviews of Geophysics, 37(2):173–199.
- ↑ Comiso, 2003, Op. cit.
- ↑ Rothrock, D.A.,Y.Yu and G.A. Maykut, 1999.Thinning of the Arctic seaice cover. Geophysical Research Letters, 26:3469–3472.
- ↑ Parkinson, C.L., D.J. Cavalieri, P. Gloersen, H.J. Zwally and J.C. Comiso, 1999. Arctic sea ice extents, areas, and trends, 1978–1996. Journal of Geophysical Research, 104(C9):20837–20856.
- ↑ Comiso, J.C., 2002. A rapidly declining perennial sea ice cover in the Arctic. Geophysical Research Letters, 29(20):1956, doi:10.1029/ 2002GL015650.
- ↑ IPCC, (2001c), Op. cit.
- ↑ IPCC, (2001b), Op. cit.
- ↑ Karoly, D.J., K. Braganza, P.A. Stott, J.M.Arblaster, G.A. Meehl, A.J. Broccoli and K.W. Dixon, 2003. Detection of a human influence on North American climate. Science, 302:1200–1203.
- ↑ Zwiers, F.W. and X. Zhang, 2003.Towards regional climate change detection. Journal of Climate, 16:793–797.
- ↑ Stott, P.A., G.S. Jones and J.F.B. Mitchell, 2003. Do models underestimate the solar contribution to recent climate change? Journal of Climate, 16:4079–4093.
- ↑ Updated from Peterson and Vose, 1997, Op. cit.
- ↑ Polyakov et al. 2002, Op. cit.
- ↑ e.g., Comiso, 2003 Op. cit.;Thompson, D.W.J. and J.M.Wallace, 1998.The Arctic Oscillation signature in the wintertime geopotential height and temperature fields. Geophysical Research Letters, 25:1297–1300.
- ↑ Serreze et al. (2000), Op. cit.
- ↑ Polyakov et al., 2002, Op. cit.
- ↑ Johannessen, O.M., L. Bengtsson, M.W. Miles, S.I. Kuzmina,V.A. Semenov, G.V. Alekseev,A.P. Nagurnyi,V.F. Zakharov, L.P. Bobylev, L.H. Pettersson, K. Hasselmann and H.P. Cattle, 2004. Arctic climate change: observed and modelled temperature and sea-ice variability. Tellus A, 56:328–341.
- ↑ Ibid.
- ↑ Goosse, H. and H. Renssen, 2003. Simulating the evolution of the Arctic climate during the last millennium. In: Proceedings of the Seventh Conference on Polar Meteorology and Oceanography and Joint Symposium on High-Latitude Climate Variations, May 12–16, 2003, Hyannis, Massachusetts, Abstract 1.5.American Meteorological Society.
- ↑ Goodison, B.E., P.Y.T. Louie and D.Yang, 1998.WMO Solid Precipitation Intercomparison, Final Report. Instruments and Observing Methods Report 67,WMO/TD 872.World Meteorological Organization, Geneva, 87pp. + Annexes.
- ↑ IPCC, (2001b), Op. cit.
- ↑ Data from the GHCN dataset, updated from Peterson and Vose, 1997, Op. cit.
- ↑ Updated from Peterson and Vose, 1997, Op. cit.
- ↑ Peterson et al., (1999), Op. cit.
- ↑ Updated from Peterson and Vose, 1997, Op. cit.
- ↑ New, M., M.Todd, M. Hulme and P. Jones, 2001. Precipitation measurements and trends in the twentieth century. International Journal of Climatology, 21:1899–1922.
- ↑ Serreze et al., 2000, Op. cit.
- ↑ Karl,T.R., R.G. Quayle and P.Ya. Groisman, 1993. Detecting climate variations and change: new challenges for observing and data management systems. Journal of Climate, 6:1481–1494.
- ↑ Mekis, E. and W.D. Hogg, 1999. Rehabilitation and analysis of Canadian daily precipitation time series. Atmosphere-Ocean, 37:53–85.
- ↑ Groisman, P.Ya. and E.Ya. Rankova, 2001. Precipitation trends over the Russian permafrost-free zone: removing the artifacts of pre-processing. International Journal of Climatology, 21:657–678.
- ↑ Groisman, P.Ya.,V.V Koknaeva,T.A. Belokrylova and T.R. Karl, 1991. Overcoming biases of precipitation: a history of the USSR experience. Bulletin of the American Meteorological Society, 72:1725–1733, updated.
- ↑ Hanssen-Bauer, I., E.J. Førland, O.E.Tveito and P.O. Nordli, 1997. Estimating regional trends – comparisons of two methods. Nordic Hydrology, 28:21–36.
- ↑ 62.0 62.1 Førland et al.<a class="external autonumber" href="index.html#endnote_62" rel="nofollow" title="#endnote_62">[136]</a> (1997)
- ↑ Hanssen-Bauer, I. and E.J. Førland, 1998. Long-term trends in precipitation and temperature in the Norwegian Arctic: can they be explained by changes in atmospheric circulation patterns? Climate Research, 19:143–153.
- ↑ Lee, S.E., M.C. Press and J.A. Lee, 2000. Observed climate variations during the last 100 years in Lapland, Northern Finland. International Journal of Climatology, 20:329–346.
- ↑ Updated from Peterson and Vose, 1997, Op. cit.
- ↑ Groisman, P.Ya., B. Sun, R.S.Vose, J.H. Lawrimore, P.H.Whitfield, E. Førland, I. Hanssen-Bauer, M.C. Serreze,V.N. Razuvaev and G.V. Alekseev, 2003. Contemporary climate changes in high latitudes of the northern hemisphere: daily time resolution. In: Proceedings of the 14th AMS Symposium on Global Change and Climate Variations. CD-ROM with Proceedings of the Annual Meeting of the American Meteorological Society, Long Beach, California, 9–13 February, 2003. American Meteorological Society, 10pp.
- ↑ Førland, E.J and I. Hanssen-Bauer, 2003. Past and future climate variations in the Norwegian Arctic: overview and novel analyses. Polar Research, 22(2):113–124.
- ↑ Førland, E.J. and I. Hanssen-Bauer, 2000. Increased precipitation in the Norwegian Arctic:True or False? Climatic Change, 46:485–509.
- ↑ Groisman et al., (2003), Op. cit.
- ↑ Sun, B., P.Ya. Groisman and I.I. Mokhov, 2001. Recent changes in cloudtype frequency and inferred increases in convection over the United States and the former USSR. Journal of Climate, 14:1864–1880.
- ↑ Groisman, P.Ya.,T.R. Karl, D.R. Easterling, R.W. Knight, P.B. Jamason, K.J. Hennessy, R. Suppiah, Ch.M. Page, J.Wibig, K. Fortuniak,V.N. Razuvaev, A. Douglas, E. Førland and P.-M. Zhai, 1999a. Changes in the probability of heavy precipitation: Important indicators of climatic change. Climatic Change, 42(1):243–283;Groisman, P.Ya., E.L. Genikhovich, R.S. Bradley and B.-M. Sun, 1999b. Trends in turbulent heat fluxes over Northern Eurasia. In: M.Tranter, R. Armstrong, E. Brun, G. Jones, M. Sharp and M.Williams (eds.). Interactions Between the Cryosphere, Climate and Greenhouse Gases, pp. 19–25. Proceedings of the International Union of Geodesy and Geophysics 1999 Symposium HS2, Birmingham, UK, July 1999. International Association of Hydrological Sciences Publication No. 256. IAHS Press.Sun et al., 2001, Op. cit.
- ↑ IPCC, (2001c), Op. cit.
- ↑ Groisman, P.Ya.,T.R. Karl, D.R. Easterling, R.W. Knight, P.B. Jamason, K.J. Hennessy, R. Suppiah, C.M. Page, J.Wibig, K. Fortuniak,V.N. Razuvaev, A. Douglas, E. Førland and P.-M. Zhai, 1998. Heavy rainfall in a changing climate. In: Proceedings of the Ninth Symposium on Global Change Studies, 11–16 January, 1998, Phoenix,Arizona, pp. j5–j10.American Meteorological Society;Groisman et al., 1999a, Op. cit.
- ↑ Akinremi, O.O., S.M. McGinn and H.W. Cutforth, 1999. Precipitation trends on the Canadian Prairies. Journal of Climate, 12:2996–3003;Mekis and Hogg, 1999, Op. cit.;Stone, D.A., A.J.Weaver and F.W. Zwiers, 2000.Trends in Canadian precipitation intensity. Atmosphere-Ocean, 38:321–347.
- ↑ 75.0 75.1 Mekis and Hogg (1999), Op. cit.
- ↑ Zhang X. et al.<a class="external autonumber" href="index.html#endnote_75" rel="nofollow" title="#endnote_75">[137]</a> (2001)
- ↑ Stone et al., (2000), Op. cit.
- ↑ Groisman, P.Ya., R.W. Knight and T.R. Karl, 2002.Very heavy precipitation over land: estimates based on a new global daily precipitation data set. In: Proceedings of the 13th Symposium on Global Change Studies, Orlando, Florida, 13–17 January, 2002, pp. 88–90.American Meteorological Society.
- ↑ Frich, P., L.V. Alexander, P. Della-Marta, B. Gleason, M. Haylock,A.M.G. Klein Tank and T. Peterson, 2002. Observed coherent changes in climatic extremes during the second half of the twentieth century. Climate Research, 19:193–212.
- ↑ Walsh, J.E.,W.L. Chapman and T.L. Shy, 1996. Recent decrease of sea level pressure in the Central Arctic. Journal of Climate, 9:480–488.
- ↑ Moritz, R.E., C.M. Bitz and E.J. Steig, 2002. Dynamics of recent climate change in the Arctic. Science, 297:1497–1501.
- ↑ Gillett, N.P., F.W. Zwiers, A.J.Weaver and P.A. Stott, 2003. Detection of human influence on sea-level pressure. Nature, 422:292–294.
- ↑ Polyakov et al., 2003b, Op. cit.
- ↑ Updated from Peterson and Vose, 1997, Op. cit.
- ↑ Groisman et al., (2003), Op. cit.
- ↑ Brown, R.D., 2000. Northern Hemisphere snow cover variability and change, 1915–97. Journal of Climate, 13:2339–2355.
- ↑ Ibid.;Cayan, D.R., S. Kammerdiener, M.D. Dettinger, J.M. Caprio and D.H. Peterson, 2001. Changes in the onset of spring in the western United States. Bulletin of the American Meteorological Society, 82:399–416;Groisman, P.Ya.,T.R. Karl and T.W. Knight, 1994. Observed impact of snow cover on the heat balance and the rise of continental spring temperatures. Science, 263:198–200.
- ↑ Vörösmarty et al., 2001, Op. cit.
- ↑ Wade, N.L., J. Martin and P.H.Whitfield, 2001. Hydrologic and climatic zonation of Georgia Basin, British Columbia. Canadian Water Resources Journal, 26:43–70.
- ↑ Groisman et al., (2003), Op. cit.
- ↑ Groisman et al., (2003), Op. cit.
- ↑ Groisman et al., (2003), Op. cit.
- ↑ Zakharov, 2003, Op. cit.
- ↑ IPCC, (2001b), Op. cit.
- ↑ Cavalieri, D.J., P. Gloersen, C.L. Parkinson, J.C. Comiso and H.J. Zwally, 1997. Observed hemispheric asymmetry in global sea ice changes. Science, 278:1104–1106.
- ↑ Johannessen, O.M., E. Shalina and M. Miles, 1999. Satellite evidence for an Arctic sea ice cover in transformation. Science, 286:1937–1939.
- ↑ Serreze et al., 2000, Op. cit.
- ↑ Parkinson, C.L., 2000. Recent trend reversals in Arctic sea ice extents: Possible connections to the North Atlantic Oscillation. Polar Geography, 24:1–12.
- ↑ Deser, C., J.E.Walsh and M.S.Timlin, 2000. Arctic sea ice variability in the context of recent atmospheric circulation trends. Journal of Climate, 13:617–633.
- ↑ Rothrock et al., 1999, Op. cit.;Wadhams, P., 1997. Ice thickness in the Arctic Ocean: the statistical reliability of experimental data. Journal of Geophysical Research, 102(C13):27951–27959.
- ↑ Holloway, G. and T. Sou, 2001. Has Arctic sea ice rapidly thinned? Journal of Climate, 15:1692–1701;Polyakov, I.V. and M.A. Johnson, 2000. Arctic decadal and interdecadal variability. Geophysical Research Letters, 27:4097–4100;Zhang T. et al., 2000
- ↑ Winsor, P., 2001. Arctic sea ice thickness remained constant during the 1990s. Geophysical Research Letters, 28(6):1039–1041.
- ↑ Brown, R.D. and P. Coté, 1992. Interannual variability of landfast ice thickness in the Canadian High Arctic, 1950–89. Arctic, 45(3):273–284;Polyakov, I.V., G.V. Alekseev, R.V. Bekryaev, U.S. Bhatt, R. Colony, M.A. Johnson,V.P. Karklin, D.Walsh and A.V.Yulin, 2003a. Long-term ice variability in Arctic marginal seas. Journal of Climate, 16(12):2078–2085.
- ↑ Kwok, R., 2000. Recent changes in Arctic Ocean sea ice motion associated with the North Atlantic Oscillation. Geophysical Research Letters, 27:775–778;Mysak, L.A., 2001. Patterns of Arctic circulation. Science, 293:1269–1270.;Polyakov and Johnson, 2000, Op. cit.
- ↑ Maslowski,W., B. Newton, P. Schlosser, A. Semtner and D. Martinson, 2000. Modelling recent climate variability in the Arctic Ocean. Geophysical Research Letters, 27(22):3743–3746.
- ↑ Gorshkov, S.G. (ed.), 1980. Atlas of the Oceans. Arctic Ocean. USSR Ministry of Defense,Voenno-Morskoy Flot., Moscow, 199pp.
- ↑ Carmack, E.C., K. Aagaard, J.H. Swift, R.W. Macdonald, F.A. McLaughlin, E.P. Jones, R.G. Perkin, J.N. Smith, K.M. Ellis and L.R. Killius, 1997. Changes in temperature and tracer distributions within the Arctic Ocean: Results from the 1994 Arctic Ocean section. Deep- Sea Research II, 44:1487–1502.Ivanov,V.V., 2000. Akademik Fedorov Cruise. Arctic Climate System Study,ACSYS Arctic Forecast, 2:2–3.Tromsø;Morison, J., M. Steele and R. Andersen, 1998. Hydrography of the upper Arctic Ocean measured from the nuclear submarine U.S.S. Pargo. Deep-Sea Research I, 45:15–38;Rudels, B., C. Darnel, J. Gunn and E. Zakharchuck, 1997. CTD observations. In: E. Rachor (ed.). Scientific Cruise report of the Arctic Expedition ARK - XI/1 of RV ‘Polarstern’ in 1995. Berichte zur Polarforschung, 226:22–25;Schauer, U., B. Rudels, E.P. Jones, L.G. Anderson, R.D. Muench, G. Bjork, J.H. Swift,V. Ivanov and A.-M. Larsson, 2002. Confluence and redistribution of Atlantic Water in the Nansen,Amundsen and Makarov basins. Annales Geophysicae, 20:257–273.
- ↑ Alekseev, G.V., L.V. Bulatov,V.F. Zakharov and V.V. Ivanov, 1998. Heat expansion of Atlantic waters in the Arctic Basin. Meteorologiya i Gidrologiya, 7:69–78.Alekseev, G.V.,Ye.I. Aleksandrov, R.V. Bekryaev, P.N. Svyaschennikov and N.Ya. Harlanienkova, 1999. Surface air temperature from meteorological data. In: Detection and Modelling of Greenhouse Warming in the Arctic and sub-Arctic, INTAS Project 97-1277,Technical Report on Task 1. Arctic and Antarctic Research Institute, St. Petersburg, Russia.
- ↑ Carmack, E.C., R.W. Macdonald, R.G. Perkin, F.A. McLaughlin and R.J. Pearson, 1995. Evidence for warming of Atlantic water in the southern Canadian Basin of the Arctic Ocean: results from the Larsen-93 expedition. Geophysical Research Letters, 22(9):1061–1064.;McLaughlin et al., 1996;Morison et al., 1998, Op. cit.
- ↑ Steele,M.and T.Boyd, 1998. Retreat of the cold halocline layer in the Arctic Ocean. Journal of Geophysical Research, 103(C5):10419–10435.
- ↑ Dickson, R.R.,T.J. Osborn, J.W. Hurrell, J. Meincke, J. Blindheim, B. Adlandsvik,T.Vinje, G. Alekseev and W. Maslowski, 2000. The Arctic Ocean response to the North Atlantic Oscillation. Journal of Climate, 13:2671–2696;Grotefendt et al., 1998)
- ↑ Dickson et al., 2000, Op. cit.
- ↑ Loeng, H.,V. Ozhigin and B. Ådlandsvik, 1997.Water fluxes through the Barents Sea. ICES Journal of Marine Science, 54:310–317.
- ↑ Alekseev, G.V., L.V. Bulatov and V.F. Zakharov, 2000. Freshwater melting/freezing cycle in the Arctic Ocean. In: E.L. Lewis, E.P. Jones, P. Lemke,T.D. Prowse and P.Wadhams (eds.).The Freshwater Budget of the Arctic Ocean, pp. 589–608. NATO Science Series, Kluwer Academic Publishers.
- ↑ e.g., Fissel, D.B. and H. Melling, 1990. Interannual variability of oceanographic conditions in the southeastern Beaufort Sea. Canadian Contractor Report of Hydrography and Ocean Sciences No 35. Institute of Ocean Sciences, Sidney, British Columbia, Canada, 102pp.
- ↑ Dickson, R.R., J. Meincke, S.-A. Malmberg and A.J. Lee, 1988.The ‘Great Salinity Anomaly’ in the Northern North Atlantic 1968–1982. Progress in Oceanography, 20:103–151.
- ↑ e.g., Hakkinen, S., 1993. An Arctic source for the Great Salinity Anomaly: a simulation of the Arctic ice-ocean system for 1955–1975. Journal of Geophysical Research, 98(C9):16397–16410.;Mysak, L.A., D.K. Manak and R.F. Marsden, 1990. Sea ice anomalies observed in the Greenland and Labrador Seas during 1901–1984 and their relation to an interceded Arctic Climate cycle. Climate Dynamics, 5:111–132.
- ↑ Alekseev et al., 2000, Op. cit.
- ↑ Steffansson, U., 1969.Temperature variation in the North Icelandic coastal area during recent decades. JoKull, 19:18–28.
- ↑ Malmberg, S.A. and J. Blindheim, 1994. Climate, cod and capelin in northern waters. ICES Marine Science Symposia, 198:297–310.
- ↑ Drinkwater, K.F., 1994. Climate and oceanographic variability in the Northwest Atlantic during 1980s and early 1990s, NAFO Scientific Council Research Document 94/71. Northwest Atlantic Fisheries Organization, Dartmouth, Nova Scotia, Canada, 39pp.
- ↑ Belkin, I.M., S. Levitus, J. Antonov and S.-A. Malmberg, 1998. Great salinity anomalies in the North Atlantic. Progress in Oceanography, 41:1–68.
- ↑ Alekseev, G.V., O.M. Johannessen, A.A. Korablev,V.V. Ivanov and D.V. Kovalevsky, 2001. Interannual variability in water masses in the Greenland Sea and adjacent areas. Polar Research, 20(2):201–208;Boenisch et al., 1997)
- ↑ Hansen, B.,W.R.Turrell and S. Østerhus, 2001. Decreasing overflow from the Nordic Seas into the Atlantic Ocean through the Faroe Bank Channel since 1950. Nature, 411:927–930.
- ↑ Zhang,T., J.A. Heginbottom, R.G. Barry and J. Brown, 2000. Further statistics on the distribution of permafrost and ground ice in the Northern Hemisphere. Polar Geography, 24(2):126–131.
- ↑ Lunardini,V.J., 1996. Climatic warming and the degradation of warm permafrost. Permafrost and Periglacial Processes, 7:311–320.
- ↑ Brown, J., K.M. Hinkel and F.E. Nelson, 2000.The Circumpolar Active Layer Monitoring (CALM) program: Research designs and initial results. Polar Geography, 24(3):165–258.
- ↑ Vourlitis, G.L. and W.C. Oechel, 1996.The heat and water budgets in the active layer of the arctic tundra at Barrow, Alaska. Journal of Agricultural Meteorology, 52:293–300.
- ↑ Ibid.
- ↑ Romanovskii, N.N. and H.W. Hubberten, 2001. Results of permafrost modelling of the lowlands and shelf of the Laptev Sea Region, Russia. Permafrost and Periglacial Processes, 12:191–202.
- ↑ Osterkamp,T.E., 2003. A thermal history of permafrost in Alaska. In: M. Phillips, S. Springman and L.U.Arenson (eds.). Permafrost, pp. 863–868. Swets & Zeitlinger, Lisse;Osterkamp,T.E. and V.E. Romanovsky, 1999. Evidence for warming and thawing of discontinuous permafrost in Alaska. Permafrost and Periglacial Processes, 10:17–37;Romanovsky,V.E. and T.E. Osterkamp, 2000. Effects of unfrozen water on heat and mass transport processes in the active layer and permafrost. Permafrost and Periglacial Processes, 11:219–239.;Romanovsky,V.E., D.O. Sergueev and T.E. Osterkamp, 2003.Temporal variations in the active layer and near-surface permafrost temperatures at the long-term observatories in Northern Alaska. In: M. Phillips, S. Springman and L.U. Arenson (eds.). Permafrost, pp. 989–994. Swets & Zeitlinger, Lisse.
- ↑ Romanovsky and Osterkamp, (2000), Op. cit.
- ↑ Jorgenson, M.T., C.H. Racine, J.C.Walters and T.E. Osterkamp, 2001. Permafrost degradation and ecological changes associated with a warming climate in central Alaska. Climatic Change, 48:551–579.
- ↑ Serreze et al., 2000, Op. cit.
- ↑ Isaksen, K., P. Holmlund, J.L. Sollid and C. Harris, 2001.Three deep alpine-permafrost boreholes in Svalbard and Scandinavia. Permafrost and Periglacial Processes, 12:13–25.