Green Revolution
Topics: |
“Green Revolution[1]” is a public relations term, probably coined in the 1960s by William Gaud, then Director of the U.S. Agency for international Development, that symbolized the modernization of agriculture in less industrialized countries by means of technological change rather than violent “Red Revolution” (Communism)[2].
The essence of the Green Revolution was developing fertilizer-responsive varieties of wheat and rice that would increase national yields of these basic cereals; however, other non-sustainable practises of farming such as overdrafting of groundwater (such as on the North China Plain) were also advanced to create short term gains, with long term detriment.
With the increased national yields, promoters of this agricultural modernization saw the potential for reducing hunger, poverty, misery, and the potential for violent social upheaval that would threaten the geopolitical interests of the United States and other western powers.
Scientific research that underlay the green revolution is generally dated from the establishment of the Mexican Agricultural Program by the Rockefeller Foundation in 1943, and the successful technological changes were well in place by the 1970s in many parts of Asia and Latin America. Green revolution technology is now almost universally the “normal” way to do agriculture in all parts of the world except in parts of Africa.
Contents
- 1 History
- 1.1 Changes in agricultural science and production
- 1.2 Food supplies and World War II
- 1.3 Reconstruction after World War II and the Cold War
- 1.4 Rockefeller and Ford Foundations create the International Rice Research Institute and the International Center for Maize and Wheat Improvement
- 1.5 New Production Practices for High Yielding Wheat and Rice
- 1.6 Primary characteristics
- 1.7 Secondary characteristics
- 2 Assessing the consequences
- 3 Further reading
- 4 References
- 5 Acknowledgments
History
Changes in agricultural science and production
For most of human evolutionary history, people were hunter-gatherers. They ate that which grew with little or no human management. Beginning about 40,000 years ago, and culminating about 10,000 years ago, however, people invented and increasingly used active management or “agriculture” to increase the quantity and security of food supplies, including grains, vegetables, and livestock. With this change, often called the “Neolithic Revolution,” modern humans became more sedentary and lived in villages or cities. A farming class tilled the soil and created the potential for large stores of grain that supported an increasingly thriving urban culture. For most of the past 10,000 years, until about 250 years ago, most (probably over 90 percent) people were tillers of the soil, and the primary human occupation was “farmer.”
With the exception of the last 100 years, the primary limitation on agricultural production was the supply of nitrogen to growing plants. The natural nitrogen cycle slowly takes the largely inert gas nitrogen (N2) from the atmosphere and fixes it into soil in forms that can be utilized as nutrients by plants.
Human activities, including the production of nitrogen fertilizers, combustion of fossil fuels, and other activities, however, have substantially increased the amounts of nitrogen fixed per year to levels that rival rates of natural nitrogen fixation. Increased levels of nitrogen fixation have already had effects on the atmosphere, terrestrial ecosystems, and aquatic ecosystems. Most of the effects are troublesome, but the still increasing uses of nitrogen fertilizer clearly have made agricultural ecosystems more productive. Nitrogen fertilizer, in a word, was essential to the productivity gains of the green revolution. As human population continues to expand, expansion of the use of nitrogen fertilizer, too, will occur[3].
Before 1900, the only major interventions people could do to increase supplies of nitrogen for crop plants lay in using manure, crop rotations with leguminous crops like clover, and mining of salts like sodium nitrate and natural materials like bird guano. These extra supplies, however, were expensive and limited in size. People were surrounded by billions of tons of inert nitrogen in the air, but it was impossible to bring this material to service of increasing yields in agriculture.
From 1909 – 1913, human intervention in the nitrogen cycle became possible on a large scale, with enormous consequences for agriculture and all human culture. This change compared to the advent of the Neolithic Revolution that changed people from nomadic hunter-gatherers to settled agriculturalists. In 1909, the German chemist Fritz Haber found a relatively inexpensive way to synthesize ammonia (NH3) from atmospheric nitrogen and hydrogen (H2). In 1913, Carl Bosch, a German chemical engineer, devised a way to scale up the Haber laboratory process to a large-scale industrial process. Thus the Haber-Bosch process enabled the making of inexpensive nitrogen fertilizer for the first time[4]. Haber won a Nobel Prize in 1918 for synthesis of ammonia, and Bosch won one in 1931 for development of chemical high pressure methods[5].
Although many new chemical products invented in the 19th and 20th centuries had strong effects on agriculture, the Haber-Bosch process for making cheap nitrogen fertilizer undoubtedly had the most profound impact. After, within 20 years of the start of large scale manufacturing of nitrogen fertilizer, the assumption was that the modern farmer used synthetic nitrogen fertilizer. To not use it was backward and inefficient.
With cheap nitrogen a possibility, farmers and agricultural scientists confronted the second limiting feature of crop plants: the genes governing the make-up of the plants. The major cereal crops (maize, rice, and wheat) had served humankind well for thousands of years, but farmers had selected seed for the next year’s crop based on the varieties that did well under conditions of limited nitrogen. These traditional varieties were perfectly serviceable and stable, and they typically yielded 0.5 – 1.0 tons per hectare per year without added nitrogen fertilizer.
Rice and wheat were particularly troublesome in the sense that if a farmer provided any extra nitrogen to these crops, they responded by “lodging.” That is, with the extra nutrients, traditional varieties grew larger grain heads, but the stems (straw) of the plants were so tall and thin that the extra weight caused the plant to collapse. The increased yields remained unavailable, because the plants fell over into the soil and were lost. In the 1920s, English wheat scientists Roland Biffen and Frank Endledow of Cambridge University stated clearly that the object of modern plant genetics was to find genetic varieties of wheat that would respond to nitrogen fertilizer without lodging[6].
Biffen and Engledow’s identification of the target for plant breeding was not widely recognized around the world, but before 1940 Chinese rice breeders and Japanese wheat breeders were doing exactly what Biffen and Engledow promoted: finding, respectively, genetic varieties of rice and wheat that increased their grain yields but did not lodge when given extra nitrogen[7]. With the work of English, Chinese, Japanese, American, and other plant breeders, the understanding of Gregor Mendel’s genetic theories of inheritance linked directly to the chemical processes invented in Germany by Haber and Bosch.
Before 1940, these new advances in plant genetics and soil fertility management remained largely at the research level, not implemented on a large scale by commercial and subsidence farmers. Use of synthetic nitrogen fertilizer began to climb steadily after 1920, but, with only a few exceptions the materials were not going to the major cereal crops: wheat and rice.
Food supplies and World War II
The outbreak of World War II, in 1937 in Asia and 1939 in Europe, created conditions leading to vastly increased interest in synthetic nitrogen and plant genetics. As with all wars, World War II severely disrupted agricultural production. Men were swept out of the fields into armies; combat prevented planting and harvesting or destroyed the yields in order to deny food to the enemy; normal trade routes, especially in wheat, fell before attacks by the enemy; governments seized control of any harvests achieved because they knew their strategic value.
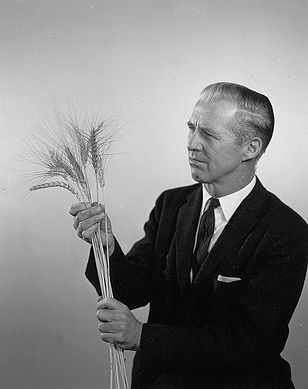
Before 1940, presidents, prime ministers, and other top leaders tended to leave the details of agricultural production to lower level leaders. In times of war, top leadership frequently confronted the question of adequacy of food supplies for the military and civilian populations. In Great Britain, for example, top leadership knew that Britain nearly lost World War I due to interruption of imported food supplies, and they had every reason to expect renewed German attacks on British food imports from North America would once again play a part in German strategy[8].
In the United States, the war-time conditions led to a particular development that, at the time, did not necessarily portend that it became a model for post-war reconstruction. The Rockefeller Foundation had run an agricultural experiment station in China since the 1920s, but the outbreak of the war and the Japanese invasion of China forced the Foundation to close up its China operations and withdraw to the western hemisphere. In 1940, the US government sought to improve its relations with neighboring Mexico, and one way of accomplishing this involved the Rockefeller Foundation establishing the Mexican Agricultural Program outside of Mexico City in 1943[9].
It probably would not have been possible for President Franklin Roosevelt to persuade Congress to allocate US government funds to a Mexican program, but high level contacts between the US government and the Rockefeller Foundation helped broker the use of Rockefeller Foundation money in Mexico.
In addition, agricultural scientists were used to working close to home, not in foreign lands. Aside from the small China operation, no one had ever thought about how a highly developed country assisted a less developed country modernize its agriculture. The Rockefeller Foundation was the only group with any experience of this sort, and they had funds no longer usable in China that could be used in Mexico.
The new Mexican president, Ávila Camacho, wanted the program, and Roosevelt also wanted better relations with Mexico. The Mexican Agricultural Program satisfied Mexico, the US, and the Rockefeller Foundation. That it became a model important to fostering the Green revolution was unforeseeable at the time it began operations.
The Mexican Agricultural Program, led by J. George Harrar, worked primarily on wheat and maize improvement. Harrar hired Norman E. Borlaug to head up the wheat improvement efforts, a choice that had far-reaching effects on the yields of wheat in Mexico and globally after the end of World War II. By dint of scientific training and personality, Borlaug became the prime scientific mover of the Green revolution.
Reconstruction after World War II and the Cold War
[10]When World War II ended in 1945, large areas of Europe and Japan lay in rubble. Food supplies were short, threatening famine in occupied Germany, and the imperial ties between Britain and British India were rapidly unraveling. With the loss of empire, Britain’s system of relying on food imports became untenable, and British India—divided into Pakistan and India—no longer had the food trading relations that existed during British rule. India’s normal supplies of wheat now lay precariously in Pakistan. To top off the new insecurity, the war-time alliance of the US-Britain-USSR devolved into a Cold War between the USSR and the US-British alliance.
The US, the only industrialized country with advanced agricultural technology not in ruins, looked at a vastly altered geopolitical context after 1945 compared to before. Under the leadership of President Harry Truman, the US committed its policy to one of containing the USSR and preventing any communist insurrection or insurgency in other countries. US leaders saw two countries as particularly susceptible to upheaval: India and the Philippines. US leaders also saw high population growth rates in these and other countries of Asia and that food supplies were not growing commensurately. Accordingly, in 1949 President Truman announced the “Point IV” program to bring aid in the form of new agricultural technology to countries like India and the Philippines.
A stumbling block of considerable significance was the fact that neither the US Department of Agriculture nor the state agricultural universities were organized or knowledgeable about how to make such technology transfer work. Into this void came the Rockefeller Foundation. Simultaneously, the newly enlarged Ford Foundation, headed by Paul Hoffman, former administrator of the Marshall Plan to reconstruct Europe, also moved vigorously to establish a Ford Foundation presence in India to promote community development and modernization.
Ultimately the Rockefeller Foundation, the Ford Foundation, and the US government provided the organizational skills, the scientific and technological vision, and the finances to create and promote the Green Revolution. Rockefeller efforts focused primarily on the scientific enterprise, Ford emphasized changing policy dimensions in recipient countries, and the United States government provided large-scale funding under the strategic umbrella of the Cold War.
Rockefeller and Ford Foundations create the International Rice Research Institute and the International Center for Maize and Wheat Improvement
Two new institutions contributed disproportionately to development of the green revolution after 1945, the International Rice Research Institute (IRRI) and the Center for the Improvement of Maize and Wheat (Spanish acronym CIMMYT). The two foundations and the government of the Philippines created IRRI in Los Baños, near Manilla in the Philippines. IRRI drew much from the Mexican Agricultural Program: intensive plant breeding to find fertilizer-responsive genetic varieties of rice, training a new scientific cadre of scientists from the less industrialized world, and promoting the production and use of new seeds producing higher yields.
IR-8 was the first of the new high yielding varieties of rice released by IRRI in 1966. IR-8 typically yielded between 4 and 7 metric tons per hectare per year, compared to 2 – 4 metric tons per hectare per year for traditional varieties. Local conditions affected yields dramatically and the yield advantage of IR8 ranged from 30 – 100 percent[11]. This was a dramatic yield increase from agricultural science.
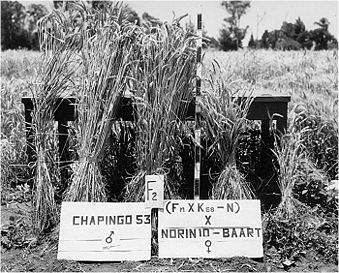
IRRI staff have variously divided the Institute’s subsequent achievements. In general, work in the 1960s focused on semidwarf varieties that achieved highly significant yield increases. This work continued and led to new varieties offering about 1% per year increases in yield from 1966 – 1996. Before 1980, most of the increase in yield was due to a higher harvest index (ratio of grain mass to mass of rest of plant); after 1980, the harvest index tended to remain about the same but the new varieties produced higher amounts of total mass and thus yields continued to increase[12]. Much of the increased yields possible with IRRI’s varieties lay in fertilizer responsiveness and photoperiod insensitivity. The latter opened the door to higher yields through multiple crops per year.
In its first years, IRRI assumed the high-energy inputs needed for high yields would be available. These included primarily energy to make the nitrogen fertilizer and energy to manage water supplies. By the 1980s, IRRI scientists became increasingly aware that, in the future, energy supplies might be more expensive or limited. In addition, the use of high amounts of energy globally was inducing climate change; that rice production under irrigation can also release methane (CH4), one of the gases that causes global warming, meant that high yielding rice cultivation made rice farming both a cause and a victim of climate change. Sustainable research objectives began to enter IRRI’s research programs[13].
Based on the enthusiasm for IRRI, the Ford and Rockefeller Foundations collaborated with the government of Mexico to form the International Center for Maize and Wheat Improvement (generally referred to by its Spanish acronym: CIMMYT) in 1966 outside Mexico City. CIMMYT replaced the Mexican Agricultural Program of the Rockefeller Foundation[14]. Like IRRI, CIMMYT emphasized intensive plant breeding to find fertilizer-responsive genetic varieties of wheat, training a new scientific cadre of scientists from the less industrialized world, and promoting the production and use of new seeds producing higher yields.
New high yielding varieties of wheat quickly became available due to earlier work of Borlaug in the Mexican Agricultural Program. Borlaug in 1953 obtained the original genetic material for high yielding wheat from Orville Vogel, a US Department of Agriculture research scientist working at Washington State College in Pullman, Washington. Vogel in 1947 had received material from Samuel Cecil Salmon, a US Department of Agriculture wheat scientist who had visited occupied, post-war Japan and found Norin 10 and other Japanese varieties that he considered quite promising. These strains contained semi-dwarfing genes that produced plants with thick, stiff straw that did not lodge under heavy nitrogen fertilization.
The first commercial varieties of high yielding varieties of wheat were grown in Washington State starting in 1961. They yielded 75 bushels per acre per year (approximately 5 metric tons per hectare) compared to traditional varieties yielding 59 bushels per acre per year (approximately 4 metric tons per hectare), a 27 percent increase. Borlaug in Mexico released the first varieties derived from Norin 10 in 1962 – 1967. These varieties increased yields from 4 – 4.5 metric tons per hectare to 6 – 6.5 metric tons per hectare[15].
Indian scientists, particularly M.S. Swaminathan at the Indian Agricultural Research Institute in New Delhi, had followed the work of Vogel and Borlaug closely, and he invited Borlaug to India in 1963. Swaminathan and other Indian scientists had known for years about the tremendous potential for higher yields of wheat under Indian conditions, but they had been unable to persuade top political leaders of the need to invest in the development of a modern Indian agriculture. This situation changed in 1964 when
Lal Badhur Shastri became Prime Minister after the death of Jawaharlal Nehru. Shastri, in contrast to Nehru, understood the fundamental importance of agriculture for development, and he moved quickly to change India’s stance on modernization. At the time he created this shift in policy, India was in the midst of two back-to-back droughts, which had created a need for heavy imports from the US. War with Pakistan in 1965 further exacerbated the sense of crisis and helped push Shastri towards the proposals from Swaminathan[16].
Borlaug visited India in 1963 and helped solidify the research program already underway at the Indian Agricultural Research Institute. High yielding wheat varieties from Mexico were well adapted to Indian conditions and immediately led to production gains: from 1 – 2.4 tons per hectare per year to 1.8 – 5.0 tons per hectare per year. As with the new semi-dwarf rice varieties, yields of traditional and the new semi-dwarf wheat varieties were highly variable depending on local conditions[17]. Nevertheless, in specific locations the yield increases were substantial, and further breeding work by Indian scientists enhanced these yields even further in the following decade.
New Production Practices for High Yielding Wheat and Rice
Thus the Green revolution emerged from a combination of scientific, technological, and geopolitical conditions. The term is generally confined to the advent of high yielding varieties of wheat and rice in less industrialized countries, but it is important to recognize that the technology of the Green revolution was primarily the development of successful varieties that were remarkably responsive to nitrogen fertilizer when water was managed properly. Those varieties now provide the vast majority of cereal grains in the world, from both the heavily industrialized countries and the less industrialized countries[18].
Primary characteristics
Fertilizer, particularly nitrogen, and water comprise the heart of the yields from the new varieties of wheat and rice. All other properties of the new varieties are secondary. The critical role of proper water management generally meant that the varieties had to be grown under irrigation. Only with irrigation could the farmer have assurance that the right amount of water would be available at the right time. Irrigation also became a reason for why new varieties, particularly of rice, were not immediately developed for all areas. Some areas are not suitable for irrigation. This lack of new varieties underlies one of the most significant criticisms of the Green revolution, as explained later.
Secondary characteristics
The new high yielding varieties of wheat and rice also had other properties affecting their growth, potential, and management. Photoperiod insensitivity is controlled by genes in both wheat and rice, and breeders found varieties in which photoperiod sensitivity was diminished or absent. Ordinarily wheat is considered a long-day plant and rice a short-day plant[19]. With photoperiod insensitivity farmers can grow the crop at any time of year (provided temperatures are appropriate. Thus photoperiod insensitivity opened the doors to multiple cropping. Instead of one or at most two crops per year, it is now possible in some areas to obtain three or even four crops per year. Thus land is utilized more intensively, and national yields rise. The productivity of any particular crop remains the same but the productivity of a hectare of land rises sharply per year.
Virtually all crop plants are damaged by insects, weeds, and plant pathogens (fungi, bacteria, and viruses). The high yielding varieties were no exception. In wheat, genetic control of fungal diseases is particularly important, and the new Mexican varieties bred by Borlaug incorporated better fungal resistance than many traditional varieties had. IR-8 bred at IRRI was susceptible to rice tungro disease, but later releases of new rice varieties incorporated better control of plant pathogens. Nevertheless this viral disease remains a problem, as are many other diseases[20]. Farmer education, particularly for insect control of rice, has been an ongoing need since the 1960s.
Mechanization frequently accompanied the introduction of high yielding varieties, but it was not always needed to obtain the higher yields. If a farmer was multi-cropping, then timeliness of harvesting, plowing, and planting frequently demanded the speed of machinery to get more crops per year. For the most part, however, mechanization was a side effect of the Green revolution: if yields and thus income went up, the farmer frequently took the extra money and invested in machinery.
Development of farm credit systems was a social innovation essential to the Green revolution. Farmers in the industrialized world borrow money at favorable interest rates to buy fertilizer, seeds, and irrigation equipment. Farmers in the less industrialized countries traditionally had no access or particular need for credit; if credit was available, it was often at high interest rates. Successful use of Green revolution technology generally required that farmers have access to credit at reasonable prices.
Green revolution technology is highly dependent upon a scientific and educational infrastructure. The new seeds required new knowledge be created and diffused throughout the countryside and in urban areas. New research needed to continue, especially for issues in pest control, to ensure that genetic resistance to pests did not succumb to further evolution of virulence in the pest organism. Now, with climate change and continuing population growth, research has even more demanding challenges to further increase yields[21].
Assessing the consequences
Controversy attended the spread of green revolution, and arguments in the 1970s were most acute. Since the 1990s, these disputes about whether the green revolution had more benefits than damages, or whether it was the right reform effort to improve human well being, have been more muted.
Benefits claimed
Without doubt, higher yields provide the main justification for claiming that the new technology was not just a success but a triumph of human ingenuity. Proponents claim that human well being was well served by the new seeds, more synthetic fertilizer, and better water management[22].
At the individual farmer level, a user of the new technology typically saw an increase in yields on his/her farm and thus had more produce to sell. Incomes rose accordingly. At the individual consumer level, i.e. a person not growing the crop but buying it for consumption, the increased supplies typically resulted in lower food prices, a benefit[23]. At the national level, governments more assured of adequate food supplies typically saw increased national and economic security[24]. Vulnerability to availability of foreign grains and vicissitudes of foreign exchange fluctuations no longer threatened famine or shortages of food.
More cereal production could, in theory, improve the quantity and quality of the food supply. In some cases, the extra production could be channeled to livestock, thus increasing the availability of meat, milk, eggs, and dairy products. Most people have considered more animal products a benefit and an improvement of diet.
More intangible, but definitely valued by urban leaders, is the notion of “modernization.” Before the green revolution in Asia and Latin America, most economies were predominantly agrarian, i.e. the major economic activity was agriculture, and most people worked tilling the soil. Modernization involves the development of a more diverse economy in which an increasing number of people leave agriculture and enter work in industry and the services. The developed countries made this transition beginning in Great Britain in the 18th century, and many countries had completed the process by the mid-20th century. In the United States, the primary transition from agrarian to industrial occurred between 1850 and 1950.
Countries still agrarian after World War II, invariably had leaders who wanted more labor to leave agriculture so as to enable the diversification and development of the economy. Subsistence, agrarian farmers, however, typically produced only enough for themselves with little surplus left over for urban markets. Only by transitioning to high yielding agriculture and urban food markets with the green revolution could the dream of modernizing the economy be realized. This transition is now well underway or near completion in countries like Mexico, India, and China, yet each of these countries was still essentially agrarian at the end of World War II. The green revolution facilitated the emergence of these new industrial powerhouses.
Damages claimed
On the other side of the assessment lie the damages that have arisen because of green revolution technology. Pollution from agrichemicals, with attendant threats to health of people, livestock, and wildlife provoke much debate. Higher uses of nitrogen fertilizer have created pollution of ground, surface, and marine waters. When fertilizers became so cheap and so effective at raising yields of cereal crops, farmers used them in increasingly high amounts. Now the waters of both industrialized and less industrialized countries typically contain plant nutrients that can directly threaten health through drinking water and create harmful algal blooms on surface or marine waters.
Pesticides have aroused particularly passionate debates. Although pesticides have typically been most heavily used on non-green revolution crops, such as fruits, vegetables, and cotton, the green revolution cereals either require or encourage higher pesticide use, particularly of herbicides for both wheat and rice and insecticides for rice. Critics have pointed out damages to health, livestock, and wildlife from these uses, including significant faunal mortality and increased risk of extinctions of numerous biota . In many cases, agricultural scientists have been able to device effective pest control alternatives based on crop genetic resistance and alternative production practices. Nevertheless, the higher yields obtainable have made it possible economically and desirable from the farmer’s viewpoint to invest more in pesticides.
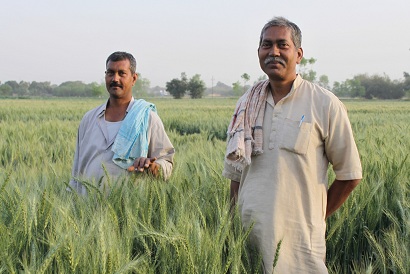
Proper water management, primarily through irrigation, was essential for expression of the fertilizer responsiveness of high yielding wheat and rice varieties. Now in numerous places around the world the intensification of water use has led to soil salinization, draw down of once abundant ground water resources, and incentives for national governments to invest heavily in dams and irrigation systems. The dams in particular have sparked intense and sometimes violent clashes between those who benefit from the new infrastructure and those who loose, mostly by being flooded out and displaced by the new reservoirs.
Biodiversity has also changed due to the Green revolution. Genetic diversity in crop plants diminished due to coordinated international research efforts based on a small selection of high yielding varieties. With narrowed genetic diversity, the crops may be more susceptible to plant disease and other pest outbreaks, which could threaten the stability of crop yields seriously. International agricultural scientists have collaborated to form seed banks to preserve as much crop genetic diversity as possible.
Affects on wild biodiversity are harder to assess. In theory, more yield from the same land area may help diminish the rate of deforestation and bringing new land into cultivation. The ensuing habitat destruction in natural savannahs and forests is the biggest threat to biodiversity on earth. In practice, however, it’s not clear that new agricultural technology really has slowed habitat destruction. Rising human population levels plus increased global affluence both stimulate settlers to migrate into the few remaining uninhabited lands of the earth, either to raise food and livestock or to procure wood products. Maybe the higher yields slowed this process, but the evidence is not strong.
Many critics have focused on the social and cultural disruption caused by the new technology and the injustices flowing from its adoption. Farmers who leased land may have lost their leases as owners took up farming their own land with the lucrative new technology. Farmers who could not adopt the new practices, due to lack of irrigation or some other factor, could be severely disadvantaged: their yields stayed the same, but the higher aggregate yields lowered the price of their harvest. Thus by not adopting, they lost income. Mechanization, a side effect of the Green revolution, may have displaced some labor, which was forced to migrate or find new work.
Urban business and political leaders may have valued the release of labor from farming as countries modernized, but a farm family forced out of its traditional livelihood undoubtedly saw the matter differently. To be uprooted from traditional culture and place and forced to migrate to cities to find work is extremely traumatic. Maybe their children will have a better life, but at least one generation becomes a technological refugee and victim.
Genetic engineering can create crops with the promise of higher yields, but sometimes the realized yields fall short of expectations, and yet the initial lure of an enhanced variety may cause a society to invest heavily in expanded agriculture; for example, newly genetically engineered soybeans tempted Argentina to conduct exptensive deforestation, resulting in considerable loss of primary forests such as the species rich Yungas Forest. Increase soybean harvests turned out to be more linked to expanded cultivation areas and not inherent yield enhancement.
Perhaps the must crushing criticism, which has emerged mostly since the 1970s, is that the Green revolution technology is unsustainable. It requires high energy, high water usage, and often brings about pollution and soil/water quality degradation. The new varieties themselves require constant updating to continue genetic resistance to pests. Without continued maintenance, the green revolution’s high yields can wither away. Continuing the practices, however, can destroy the very resources needed to achieve the yields.
Can these practices continue indefinitely for future generations, or has the new, modern agriculture sown the seeds of its own destruction and collapse? No empirical evidence is ever possible to answer this question: we can only wait and see. In the meantime, the green revolution technology feeds a steadily growing human population and demands continuing scientific and technological refinement. If one has faith in this outpouring of human ingenuity, one can predict sustainability. Without the faith, the prediction is otherwise.
Further reading
- Anderson, Robert S., Edwin Levy, and Barrie M. Morrison, Rice Science and Development Politics: Research Strategies and IRRI’s Technologies Confront Asian Diversity (1950 – 1980) (Oxford: Oxford University Press, 1991), 394 pp.
- Borlaug, Norman E., Feeding a world of 10 billion people: the miracle ahead, In Vitro Cellular Developmental Biology—Plant 38 (March – April, 2002): 221 – 228.
- David, Christina C. and Keijiro Otsuka, eds., Modern Rice Technology and Income Distribution in Asia (Boulder: L. Riener Publishers, 1993), 475 pp.
- Griffin, Keith B., The Political Economy of Agrarian Change: An Essay on the Green Revolution (Cambridge, MA: Harvard University Press, 1974), 264 pp.
- Perkins, John H., Geopolitics and the Green Revolution: Wheat, Genes, and the Cold War (New York: Oxford University Press, 1997), 337 pp.
- Shiva, Vandana, The Violence of the Green Revolution: Third World Agriculture, Ecology, and Politics (London: Zed Books, 1991), 264 pp.
- Smil, Vaclav, Enriching the Earth: Fritz Haber, Carl Bosch, and the Transformation of World Food Production (Cambridge, MA: MIT Press, 2001), 338 pp.
References
Acknowledgments
I'm grateful to Eloise Phipps and Mike Listman of CIMMYT and to Chrisanto Quintana and Sophie Clayton of IRRI for assistance in locating photos illustrating this article. I also thank Peter Saundry of NCSE for excellent editorial advice.