Climate change and insects as a forest disturbance in the Arctic
This is Section 14.8 of the Arctic Climate Impact Assessment.
Lead Author: Glenn P. Juday; Contributing Authors: Valerie Barber, Paul Duffy, Hans Linderholm, Scott Rupp, Steve Sparrow, Eugene Vaganov, John Yarie; Consulting Authors: Edward Berg, Rosanne D’Arrigo, Olafur Eggertsson,V.V. Furyaev, Edward H. Hogg, Satu Huttunen, Gordon Jacoby, V.Ya. Kaplunov, Seppo Kellomaki, A.V. Kirdyanov, Carol E. Lewis, Sune Linder, M.M. Naurzbaev, F.I. Pleshikov, Ulf T. Runesson,Yu.V. Savva, O.V. Sidorova,V.D. Stakanov, N.M.Tchebakova, E.N.Valendik, E.F.Vedrova, Martin Wilmking.
Contents
Role of insects in the boreal forest (14.8.1)
The boreal forest is naturally subject to periodic large-scale tree mortality from insect outbreaks. The death of attacked trees has a considerable influence on the balance of carbon sequestered by forests. In boreal forests of Canada, insect-caused timber losses (tree death) may be up to 1.3-2.0 times greater than the mean annual losses due to fires[1]. The particular insects involved in large-scale boreal outbreaks include bark and wood-boring beetles, defoliating insects (often Lepidoptera – moths and butterflies), and insects that attack roots and cones. Major population increases of these insects (irruptions), along with wildland fires, are the major short-term agents of change in the boreal forest. Insect-caused tree mortality can appear suddenly and within 1-10 years affect hundreds of thousands to millions of hectares (ha) of boreal forest in an outbreak, or related series of outbreaks. Moderate to severe defoliation of trees by insects has affected nearly 16 million ha/yr in the province of Ontario since 1975[2]. Nearly all boreal forest regions are subject to this phenomenon, and it may represent an adaptive response in which simultaneous mass tree death helps create conditions for the renewal of site productivity through processes that warm soils, mobilize nutrient elements, and promote simultaneous tree regeneration.
The peculiar vulnerability of boreal forests to large-scale insect disturbance may also be related to the low biodiversity of both the host tree populations (limited number of species) and the limited diversity within the complex of tree-attacking insects and the populations of predators and parasites that work to stabilize their numbers. Ecological theory predicts that systems with few species should be less stable or buffered against major population swings, and of the major forest regions of the world, the boreal forest is the least species-rich in most taxonomic groups. Finally, the occurrence of tree hybrids may be a non-climatic risk factor for large-scale insect outbreaks. Where the distributions of two wind-pollinated tree species of the same genus (e.g., spruce, pine, larches) approach each other or overlap, the trees can interbreed and form hybrid offspring. Because the hybrid trees contain a random assortment of the genes of both parents, they seldom inherit highly integrated and complete defense traits against insect attacks, such as specific chemical defenses or timing of events to avoid vulnerability. As a result, tree defenses against insect attack may be lowest in hybrid zones[3].
The level of tree mortality caused by insect outbreaks can vary from selective removal of a few percent of dominant trees to the death of nearly the entire forest canopy. Although vulnerable tree species and age classes must be present for insect outbreaks to occur, climatic events are often the trigger or proximate cause of the insect population increases and associated widespread tree mortality. In general, many organisms in the boreal region, and especially the northern boreal forest region, are heat-limited, that is, they could perform (survive, grow, and reproduce) better with more warmth if other factors did not become limiting. Many insect agents of tree mortality in the northern boreal region are heat-limited, so that sustained periods of abnormally warm weather are often associated with irruptions of insects that attack boreal tree species. Given that this is a natural climate-driven system, it is important to distinguish climate change effects from natural operation of this system.
Generally, a climate change effect imposed on the natural insect/tree-death system is very likely to result in a greater frequency of insect outbreaks; more extensive areas of tree mortality during outbreaks; and greater intensity of insect attack resulting in higher average levels of tree death within outbreak areas. The sustained operation of these factors as a result of temperature increases, if they occur, is very likely to begin to change regional tree species composition within decades. A necessary confirmation of the occurrence of a specific climate change effect on the insect/tree-death process would include the detection of differences between the composition of the new, altered forest and historical and recent paleo-ecological records of previous generations of forest. An alternative possibility that needs to be considered is that temperature increases (along with increased precipitation) will possibly produce a more favorable growth environment for certain tree species on certain site types. The increased vigor of the trees is very likely to increase their ability to produce defensive compounds and successfully resist the similarly likely increased levels of insect attacks.
It is possible that the influences of climate warming on forests and on tree-hosted insects (positive and negative for forest health) could occur simultaneously in different locations of the boreal forest, or could occur sequentially in one place at different magnitudes and rates of temperature increase. As a result, temperature increases could be associated with improved forest health in some locations and forest health problems elsewhere, and the climate change effect at any given time would be the sum of these two outcomes. In addition, the initial stage of temperature increases would produce one profile of the balance of forest health outcomes across the area of analysis, but later stages representing greater temperature increases would shift the previous balance of forest health outcomes. The empirical record strongly suggests overall negative effects on boreal forest health from sustained temperature increases.
Spruce bark beetle in Alaska (14.8.2)
During the 1990s, the Kenai Peninsula in south-central Alaska experienced the largest outbreak of spruce bark beetles (Dendroctonus rufipennis) in the world[4]. The forests of the Kenai lowlands are transitional between coastal Sitka spruce (Picea sitchensis) rainforests and the white spruce–paper birch boreal forests of semiarid central Alaska. From 1989 to 1999, more than 1.6 million ha of mature forest made up of white spruce and hybrids of Sitka and white spruce (called Lutz spruce) in south-central Alaska experienced at least 10 to 20% tree mortality, the threshold level for aerial mapping detection. In much of the insect outbreak area, virtually all mature canopy trees were killed. Extensive logging was initiated to salvage beetle-killed timber on private lands, and the US federal government has found it necessary to appropriate funds to reduce fuel hazards in stands with numerous dead trees after infestation.
The first documented spruce bark beetle infestation on the Kenai Peninsula occurred in 1950. Local infestations occurred throughout the 1950s and 1960s on the northern Peninsula[5]. A major outbreak in the northern Peninsula that started in 1970 prompted the US Forest Service to initiate annual surveys in 1971, which have continued with few interruptions. Annual survey maps show that spruce bark beetles are endemic to Kenai Peninsula forests, at least under the present climatic regime. In any given year, there is always a background level of infestation somewhere in south-central Alaska, with periodic major outbreaks in some years.
The relationship of spruce bark beetle to climate involves two direct temperature controls over [[population]s] of the insect, and an indirect control through host tree resistance. Two successive cold winters depress the survival rate of spruce bark beetle to such a low level that there is little outbreak potential for the following season[6]. The spruce bark beetle is a heat-limited organism on the Kenai Peninsula and normally requires two summers to complete its life cycle. During abnormally warm summers on the Kenai Peninsula, the spruce bark beetle can complete its life cycle in one year, dramatically increasing the potential for population buildup[7]. Tree health increases host resistance to beetle attack: healthy spruce trees can successfully resist moderate numbers of beetle attacks by opposing the wood-boring activity of females entering the tree to lay eggs with pitch under high turgor pressure[8]. The beetles are unable to overcome the flow of pitch and either are expelled or succumb in their pitch tubes. Host trees that are under stress, including either climate stress or stress from mechanical breakage, have reduced growth reserves, less pitch, and lower turgor pressure, and so are less able to resist spruce bark beetle attacks. When regional events stress entire populations of trees, spruce bark beetle reproductive success is greatly increased.
The US Forest Service conducts annual aerial surveys for “red needles” (i.e., conifer needles dead for a year or less) that allow areas of spruce killed by spruce bark beetle within the previous year to be mapped. These annual surveys describe the areal extent of active infestation; densities of recently killed trees can range from a few stems to hundreds of stems per hectare[9]. There is a one- to three-year lag between beetles entering a stand and a standwide flush of red needles (tree death). Typically, a sequence of red-needle acreage maps on the Kenai Peninsula will show a rise and fall of active infestation over a 5-10 year period on a scale of 100-1,000 ha. After that time, nearly all white/Sitka/Lutz spruce greater than 12 centimeters (cm) in diameter will generally be dead in the affected stand, and subsequent surveys will record no more red-needle acreage for that stand.
Following the 1976–1977 increase in North Pacific sea surface [[temperature]s][10], mean annual and summer temperatures in the Kenai Peninsula lowlands rose 0.5-1.5 °C. This temperature increase is the latest acceleration of post-LIA (Little Ice Age) climate warming in south-central Alaska[11]. Until recently, the most visible manifestation of the late 20th-century warming was a drying landscape, with dried-up ponds, falling water levels (as much as 1 meter [m]) in closed-basin lakes, and black spruce invasion of peat wetlands across the landscape.
Annual red-needle acreages for the southern Kenai Peninsula can be compared with standardized climate parameters recorded at the Homer Airport climate station (Fig. 14.26). In Fig. 14.26c, the drought index is calculated by subtracting the normalized past October to present September total precipitation from the normalized May through August mean temperature of the named year. The drought index is designed to combine the moisture contribution of the previous winter with the growing season moisture and temperature of the identified year. The first major spruce bark beetle outbreak of the post-1950 period occurred in the early 1970s, following the extremely warm and dry period of 1968 to 1969 on the northern Peninsula (Fig. 14.26). Red-needle mortality dropped to nearly zero by 1975, following three cool summers (1973–1975). On both the northern and southern Peninsula, the sustained onset of warm summers beginning in 1987 was followed by substantial increase in red-needle mortality beginning in 1990 and reaching a maximum in 1996. Annually mapped red-needle area declined after 1996 on the southern Peninsula because beetles had killed most of the available mature spruce forest, not because climatic factors became less favorable for insect population increases.
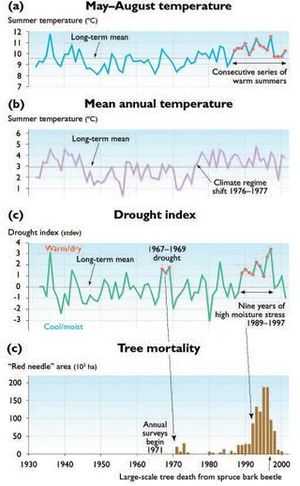
The association of red-needle mortality with drought is particularly striking in the southern Peninsula forests between 1989 and 1997 (Fig. 14.26c,d). Spruce bark beetles first attack the large, slowly growing trees in a stand[13]. The survival of smaller trees (both young saplings and released understory pole-size trees) is attributed to their ability to produce enough pitch to swamp the beetle galleries and kill the larvae. An additional factor may be that large trees are more prone to drought stress. Due to cold soils, spruce trees in Alaska usually root within the upper 30-40 cm of the soil and extend their roots laterally for 1-2 m. This shallow, extended rooting can bring trees into competition with neighboring trees and surface vegetation, especially in times of drought. Ring widths in many large trees generally decline for 5-10 years prior to the tree succumbing to bark beetles, clearly signifying a stressed condition. Drought is the most likely source of this stress[14] (Fig. 14.26).
While warm summers are the proximate cause of outbreaks, spruce stands must reach a certain level of maturity before serious spruce bark beetle infestation can develop, regardless of summer [[temperature]s]. For example, relatively light levels of tree mortality from bark beetles occurred in an area near the headquarters of the Kenai National Wildlife Refuge that burned in 1926, while a nearby stand of spruce 125 years old or older experienced heavy mortality. Many stands on the southern Kenai Peninsula were composed of trees that regenerated following a moderate spruce bark beetle outbreak in the 1870s and 1880s, and those trees only matured to a condition that would support substantial spruce bark beetle infestations in the mid-20th century. By the time that warm summers began in 1987, these trees had once again become prime bark beetle habitat.
Moisture appears to be another important factor in spruce bark beetle outbreaks on the Kenai Peninsula. Precipitation at the Kenai Airport station reached a record low from 1967 to 1969, and was at a near-record low (to that date) at the Homer Airport station during that period. This dry period was followed by an intense but short-lived spruce bark beetle outbreak from 1970 to 1974 (Fig. 14.26c,d). It appears that at least two warm summers are required to initiate a spruce bark beetle outbreak. This is consistent with the life cycle of the beetles, which normally takes two years to complete, except in the warmest summers when it can be completed in one year[15]. In 1987, summer temperatures shifted above the mean (Fig. 14.26a), beginning a sustained series of warm summers that is unique in both the instrumental and local reconstructed temperature records. After 1987, the spruce bark beetle outbreak accelerated, and no period of several cool summers, which could thermally arrest the outbreak, has occurred to the present. Red-needle acreage, however, has dropped steadily since 1996 because most of the mature spruce forest has been killed. Food supply, rather than climate, appears to have been the ultimate limiting factor in this outbreak, unlike outbreaks during the past 200-250 years studied by staff of the Kenai National Wildlife Refuge.
These results suggest that there is a continuing high risk from climate change to the management of forest land in south-central Alaska for spruce forest crops. Under recent climate conditions, and especially under scenarios of further temperature increases, spruce bark beetle irruption potential is very likely to remain high. As the small surviving (understory) spruce trees in the region mature to commercial forest dimensions, they will move into the prime size and age classes to serve as hosts for spruce bark beetle. Under these circumstances, the regional environment is very likely to remain effectively saturated with spruce bark beetles because climate limitations on beetles have been removed. Investments in regeneration and early tending of new commercial stands of spruce, should that be desired, would carry considerable risk because bark beetles would become effective agents of tree mortality at about the time that stands of spruce became large enough to generate commercial value.
Spruce budworm in North America (14.8.3)
The eastern spruce budworm (Choristoneura fumiferana), is a defoliating insect affecting conifer trees, generally in the southern and central boreal region of the United States and Canada. The absolute distribution limits of spruce budworm generally follow that of white spruce throughout the boreal forest, and there are records of severe defoliation within 150 km of the Arctic Circle[16]. Outbreaks have at times extended over 72 million ha and lasted for up to 15 years[17]. The budworm is generally present in the forest at low background numbers. During irruptions, there can be more than 22 million budworm larvae per hectare in suitable forest stands[18].
Weather appears to be a critical factor in determining budworm distribution. Irruptions of budworm generally follow drought, and outbreaks also start after hot, dry summers[19]. Drought stresses the host tree population, reducing host resistance[20]. Elevated summer [[temperature]s] increase budworm reproductive output. Female budworms lay 50% more eggs at 25 °C than at 15 °C[21]. Finally, higher temperatures and drought can shift the timing of budworm reproduction so that natural parasitoid predators are no longer effective in limiting budworm numbers[22]. Conversely, cold weather can stop a budworm outbreak. Budworms starve if a late spring frost kills the new shoot growth of the host trees on which the larvae feed.
Given this weather/climate sensitivity of budworm, it was inferred that a warming climate would be associated with northward movement of spruce budworm outbreaks[23]. Such a northward movement appears to have happened. Before about 1990, spruce budworm had not appeared able to reproduce in the northern boreal forest of central Alaska. In that year, after a series of warm summers, a spruce budworm irruption occurred in the Bonanza Creek Long-Term Ecological Research site and visible canopy damage spread over tens of thousands of hectares of nearby white spruce forest. Populations of budworm have persisted in this area near the Arctic Circle, including a minor outbreak in 2002–2003.
Other forest-damaging insects in North America (14.8.4)
Aspen is the most important deciduous tree species in the Canadian boreal forest, with more than 1,000 Tg of carbon stored in the aboveground biomass. Aspen dieback has become conspicuous over parts of the southern boreal forest and aspen parkland in western Canada. In 18 aspen stands near Grande Prairie, Alberta, defoliation histories were reconstructed based on tree rings and records of past insect outbreaks. Several factors contributed to the observed dieback. Defoliation by the forest tent caterpillar (Malacosoma disstria) and drought in the 1960s and 1980s led to reduced growth and predisposed some stands to secondary damage by wood-boring insects and fungal pathogens. Thaw–freeze events during a period of unusually light late winter snow cover (1984–1993) also contributed to the observed dieback. Under climate warming, the severity of these stressors is very likely to increase, which would pose a serious concern for the future health, productivity, and carbon sequestration of aspen forests in the region[24].
During the last two decades of the 20th century, warmer summers and winters in central Alaska were associated with a noticeable increase in the area of trees killed or damaged by insects. The mapped area of forest affected by spruce budworm, spruce coneworm (Dioryctria reniculelloides), and larch sawfly (Pristiphora erichsonii) defoliation mapped throughout Interior Alaska increased, totaling over 300,000 ha of combined infestations during the period 1991 to 1996[25].
Various insects are known have the potential to become serious agents of tree mortality in a much warmer boreal and arctic region. Insect species not currently present are almost certain to be able to disperse readily into the region if higher [[temperature]s] allow, and some are likely to develop outbreak potential. In Ontario, Canada, forest land managers estimate that a range of forest-damaging insects that are currently prominent in the central and southern boreal area would move northward or, if already present, develop greater outbreak potential[26]. These include spruce budworm, forest tent caterpillar, jack pine budworm (Choristoneura pinus pinus), and gypsy moth (Lymantria dispar). The bronze birch borer (Agrilus anxius) is a North American species that can cause severe damage to paper birch and may be effective in limiting the survival of birch along the southern (warm) margin of its distribution[27]. The bronze birch borer is present today in small numbers as far north as Alaska. The ACIA(Arctic Climate Impact Assessment)-designated models project temperatures in northern boreal North America near the temperatures of areas where the bronze birch borer effectively limits birch today. In boreal Alaska, a larch sawfly outbreak killed most of the larger and older tamarack (Larix laricina) trees during a warm period in the decade of the 1990s, and aspen leaf miner (Phyllocnistis populiella) appeared at outbreak levels (142,000 ha) by 2003[28].
Tree-damaging insects in northern Europe (14.8.5)
Several insect species with tree hosts in northern European boreal forests regularly undergo population outbreaks, and most have some connection to direct or indirect effects of warm weather anomalies and temperature limitation. In Europe, temperature increases, especially in the form of warmer winters, are associated with increased outbreaks of various species of bark beetles and aphids[29]. Bark beetles (Coleoptera, Scolytidae) are present in the boreal region of northern Europe. A key feature of temperature sensitivity is the timing of the first flight of mature adults in the spring as they seek new host trees to attack. Many beetles do not begin spring flight until air temperature reaches a threshold level. The bark beetle Ips typographus (spruce engraver beetle) built up in numbers in trees damaged by heavy storm and snow damage in the late 1960s, and then broke out in large numbers during a series of warm years in the early 1970s[30]. A similar combination of widespread tree host susceptibility and warm summers in the future is very likely to result in similar outbreaks and tree death. There are no examples in Europe of bark beetles simultaneously expanding their distribution in one direction and retracting in another[31].
Tree-defoliating insects that are responsive to temperature increases are capable of causing large-scale tree death and injury in the northernmost forests of Europe[32]. Geometrid moths of two species occur in the mountain birch (Betula pubescens ssp. czerepanovii) forest that makes up the ecotone between boreal forest and the tundra of Fennoscandia. The autumnal moth (Epirrita autumnata) and the winter moth (Operophtera brumata) regularly reach outbreak levels. Although both species lay eggs on a variety of trees and shrubs, the main host is mountain birch. Outbreaks of the autumnal moth mainly occur in mature forests in inland locations, whereas winter moth outbreaks are restricted to warm south-facing slopes or warmer locations along the Norwegian coast[33].
Populations of both the winter and autumnal moth fluctuate from low to high levels with 7 to 12 years between peak densities[34]. Severe defoliation causes death of older stems, which usually triggers vigorous sprouting of new stems from the base of the tree. However, the recovery process in these forests is slow, and may take about a century[35]. Particularly severe defoliation may kill the underground portions of the tree as well. The natural cyclic fluctuation of geometrid moth numbers is driven by density-dependent factors that lag outbreaks in time, including buildup of natural enemies, disease, and reduced food quality, and by weather conditions at all life stages. As do many of the macrolepidoptera, autumnal moths overwinter as eggs, making them vulnerable to extreme cold [[temperature]s] that reduce survival, particularly egg masses placed in the tree canopy above the snow limit. From 1960 to 1990, minimum winter temperatures below -36 °C, along with fine-scale patches of trees on dry and nutrient poor soils, efficiently predicted the distribution of autumnal moth outbreaks in birch forests of Finland[36]. Climate change involving an increase in minimum winter temperatures is likely to increase the frequency, and possibly the severity, of outbreaks of this species and others that overwinter as eggs[37]. Warmer summer temperatures with no change in winter temperatures are likely to reduce the geometrid moth outbreak potential, because higher densities of moth predators associated with warm summers would partially protect birch, while the winter limitation on the moths would still operate. Finally, if climate change consists of warmer summers and winters, as projected by the ACIA-designated models, current insect population/ecological models are not adequate to project overall effects on outbreaks[38], although new insect distributions and novel climate limitations and outbreak patterns are very likely under such circumstances (see also ACIA, Section 7.4.1.4).
A diprionid sawfly (Neodiprion sertifer) is the most serious defoliator of pine forests in northern Europe. Outbreaks mostly occur on dry and infertile sites. Minimum winter [[temperature]s] below -36 °C also limit this species. Outbreaks in Finland have been most frequent in southern and central inland areas, and temperature increases are likely to make outbreaks more common in eastern and northern areas[39].
Chapter 14: Forests, Land Management, and Agriculture
14.1. Introduction (Climate change and insects as a forest disturbance in the Arctic)
14.2. The boreal forest: importance and relationship to climate
14.3. Land tenure and management in the boreal region
14.4. Use and evaluation of the ACIA scenarios
14.5. Agriculture (Agriculture in the Arctic)
14.6. Tree rings and past climate
14.7. Direct climate effects on tree growth
14.8. Climate change and insects as a forest disturbance
14.9. Climate change and fire
14.10. Climate change in relation to carbon uptake and carbon storage
14.11. Climate change and forest distribution
14.12. Effects of ultraviolet-B on forest vegetation
14.13. Critical research needs
References
Citation
Committee, I. (2012). Climate change and insects as a forest disturbance in the Arctic. Retrieved from http://editors.eol.org/eoearth/wiki/Climate_change_and_insects_as_a_forest_disturbance_in_the_Arctic- ↑ Volney, W.J. A. and R. A. Fleming, 2000. Climate change and impacts of boreal forest insects. Agriculture, Ecosystems and Environment, 82(1–3):283–294.
- ↑ Parker, W.C., S.J. Colombo, M.L. Cherry, M.D. Flannigan, S. Greifenhagen, R.S. McAlpine, C. Papadopol and T. Scarr, 2000. Third millennium forestry: what climate change might mean to forests and forest management in Ontario. The Forestry Chronicle, 76(3):445–461.
- ↑ Whitham, T.G., 1989. Plant hybrid zones as sinks for pests. Science, 244:1490–1493.–Whitham, T.G., P. A. Morrow and B.M. Potts, 1994. Plant hybrid zones as centers of biodiversity: The herbivore community of two endemic Tasmanian eucalypts. Oecologia, 97:481–490.
- ↑ Werner, R. A., 1996. Forest health in boreal ecosystems of Alaska. The Forestry Chronicle, 72(1):43–46.
- ↑ Holsten, E.H., 1990. Spruce Beetle Activity in Alaska: 1920–1989.
- ↑ Ibid.
- ↑ Werner, R. A. and E.H. Holsten, 1985. Factors influencing generation times of spruce beetles in Alaska. Canadian Journal of Forest Research, 15:438–443.
- ↑ Holsten, E.H., 1990. Spruce Beetle Activity in Alaska: 1920–1989.
- ↑ USDA Forest Service, 1950–1999. Forest Insect and Disease Conditions in Alaska. Annual Reports. U.S. Department of Agriculture, Forest Health Protection.
- ↑ Mantua, N.J., S.R. Hare, Y. Zhang, J.M. Wallace and R.C. Francis, 1997. A Pacific interdecadal climate oscillation with impacts on salmon production. Bulletin of the American Meteorological Society, 78:1069–1079.
- ↑ Jacoby, G.C. and R.D. D’Arrigo, 1995. Tree ring width and density evidence of climatic and potential forest change in Alaska. Global Biogeochemical Cycles, 9:227–234.–Wiles, G.C., R.D. D’Arrigo and G.C. Jacoby, 1996. Summer temperature changes along the Gulf of Alaska and the Pacific Northwestern coast modeled from coastal tree rings. Canadian Journal of Forest Research, 26:474–481.
- ↑ E. Berg, 2002. pers. comm. Kenai National Wildlife Refuge, Soldotna, Alaska.–NOAA, 2002. Daily Climatological Data, Summary of the Day (TD-3200). U.S. Department of Commerce, National Oceanic and Atmospheric Administration, National Climatic Data Center, Asheville.–Wittwer, D., 2004. Forest Health Conditions in Alaska, 2003. USDA Forest Service General Technical Report R10-TP-123, 82p.
- ↑ Hard, J.S., 1985. Spruce beetles attack slowly growing spruce. Forest Science, 31:839–850.–Hard, J.S., 1987.Vulnerability of white spruce with slowly expanding lower boles on dry, cold sites to early seasonal attack by spruce beetles in south central Alaska. Canadian Journal of Forest Research, 17:428–435.
- ↑ Hard, J.S., 1987.Vulnerability of white spruce with slowly expanding lower boles on dry, cold sites to early seasonal attack by spruce beetles in south central Alaska. Canadian Journal of Forest Research, 17:428–435.
- ↑ Werner, R. A. and E.H. Holsten, 1985. Factors influencing generation times of spruce beetles in Alaska. Canadian Journal of Forest Research, 15:438–443.
- ↑ Harvey, G. T., 1985. The taxonomy of the coniferophagous Choristoneura (Lepidoptera Tortricidae): a review. In: C.J. Sanders, R. W. Stark, E.J. Mullins and J. Murphy (eds.). Recent Advances in Spruce Budworms Research, pp. 16–48. Canadian Forestry Service.
- ↑ Fleming, R. A. and W.J. A. Volney, 1995. Effects of climate change on insect defoliator population processes in Canada’s boreal forest: some plausible scenarios. Water, Soil, and Air Pollution, 82:445–454.
- ↑ Crawford, H.S. and D. T. Jennings, 1989. Predation by birds on spruce budworm Choristoneura fumiferana: functional, numerical, and total responses. Ecology, 70:152–163.
- ↑ Fleming, R. A. and W.J. A. Volney, 1995. Effects of climate change on insect defoliator population processes in Canada’s boreal forest: some plausible scenarios. Water, Soil, and Air Pollution, 82:445–454.
- ↑ Mattson, W.J. and R. A. Haack, 1987. The role of drought in outbreaks of plant-eating insects. BioScience, 37:110–118.
- ↑ Jardine, K., 1994. Finger on the carbon pulse. The Ecologist, 24:220–224.
- ↑ Mattson, W.J. and R. A. Haack, 1987. The role of drought in outbreaks of plant-eating insects. BioScience, 37:110–118.
- ↑ Gitay, H., S. Brown, W. Easterling and B. Jallow, 2001. Ecosystems and their goods and services. In: J.J. McCarthy, O.F. Canziani, N. A. Leary, D.J. Dokken and K.S. White, (eds.). Climate Change 2001: Impacts, Adaptation, and Vulnerability. Contribution of Working Group II to the Third Assessment Report of the Intergovernmental Panel on Climate Change, pp. 235–342. Cambridge University Press.
- ↑ Hogg, E.H., J.P. Brandt and B. Kochtubajda, 2002. Growth and dieback of aspen forests in northwestern Alberta, Canada in relation to climate and insects. Canadian Journal of Forest Research, 32:823–832.
- ↑ Holsten, E. and R. Burnside, 1997. Forest health in Alaska: an update. Western Forester, 42(4):8–9.
- ↑ Parker, W.C., S.J. Colombo, M.L. Cherry, M.D. Flannigan, S. Greifenhagen, R.S. McAlpine, C. Papadopol and T. Scarr, 2000. Third millennium forestry: what climate change might mean to forests and forest management in Ontario. The Forestry Chronicle, 76(3):445–461.
- ↑ Haak, R. A., 1996. Will global warming alter paper birch susceptibility to bronze birch borer attack? In: W.J. Mattson, P. Niemila and M. Rossi (eds.). Dynamics of Forest Herbivory: Quest for Pattern and Principle, pp. 234–247.
- ↑ Wittwer, D., 2004. Forest Health Conditions in Alaska, 2003. USDA Forest Service General Technical Report R10-TP-123, 82p.
- ↑ Beniston, M., R.S.J. Tol, R. Delecolle, G. Hoermann, A. Inglesias, J. Innes, A.J. McMichael, W.J.M. Martens, I. Nemesova, R. Nicholls and F.L. Toth, 1998. Europe. In: R. T. Watson, M.C. Zinyowera, R.H. Moss and D.J. Dokken (eds.). The Regional Impacts of Climate Change: An Assessment of Vulnerability, pp. 151–185. A Special Report of Working Group II of the Intergovernmental Panel on Climate Change. Cambridge University Press.
- ↑ Heliövaara, K. and M. Peltonen, 1999. Bark beetles in a changing environment. Ecological Bulletins, 47:48–53.
- ↑ Ibid.
- ↑ Bylund, H., 1999. Climate and the population dynamics of two insect outbreak species in the north. Ecological Bulletins, 47:54–62.–Danell, Kj., A. Hofgaard,T.V. Callaghan and J.B. Ball, 1999. Scenarios for animal responses to global change in Europe’s cold regions: an introduction. Ecological Bulletins, 47:8–15.
- ↑ Neuvonen, S., P. Niemalä and T.Virtanen, 1999. Climatic change and insect outbreaks in boreal forests: the role of winter temperatures. Ecological Bulletins, 47:63–67.
- ↑ Altenkirch,W., 1990. Zyklische Fluktuation beim Kleinen Frostspanner (Operophtera brumata). Allgemeine Forst- und Jagdzeitung, 162:2–7.
- ↑ Bylund, H., 1999. Climate and the population dynamics of two insect outbreak species in the north. Ecological Bulletins, 47:54–62.
- ↑ Neuvonen, S., P. Niemalä and T.Virtanen, 1999. Op cit.
- ↑ Ibid.
- ↑ Ibid.
- ↑ Ibid.