Implications of current species distributions for future biotic change in the Arctic
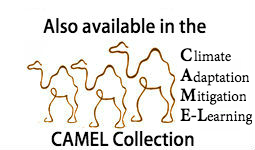
This is Section 7.3.1 of the Arctic Climate Impact Assessment Lead Author: Terry V. Callaghan; Contributing Authors: Lars Olof Björn, F. Stuart Chapin III,Yuri Chernov,Torben R. Christensen, Brian Huntley, Rolf Ims, Margareta Johansson, Dyanna Jolly Riedlinger, Sven Jonasson, Nadya Matveyeva,Walter Oechel, Nicolai Panikov, Gus Shaver; Consulting Authors: Josef Elster, Heikki Henttonen, Ingibjörg S. Jónsdóttir, Kari Laine, Sibyll Schaphoff, Stephen Sitch, Erja Taulavuori, Kari Taulavuori, Christoph Zöckler
Contents
Plants (7.3.1.1)
Species diversity
About 3% (~5900 species) of the global flora occurs in the Arctic as defined in this chapter (0.7% of the angiosperms (flowering plants), 1.6% of the gymnosperms (cone-bearing plants), 6.6% of the bryophytes, and 11% of the lichens) (Table 7.2).There are more species of primitive taxa (cryptogams), that is, mosses, liverworts, lichens, and algae, in the Arctic than of vascular plants (Matveyeva and Chernov, 2000). Less than half (about 1800) of arctic plant species are vascular plants.There are about 1500 species of vascular plants common to both Eurasia[1] and North America[2]. Similar numbers of non-vascular plants probably occur in the Arctic on both continents, although their diversity has been less thoroughly documented. In the Russian Arctic, for example, 735 bryophyte species (530 mosses and 205 liverworts) and 1078 lichen species have been recorded[3]. In general, the North American and Eurasian Arctic are similar to one another in their numbers of vascular and non-vascular plant species, of which a large proportion (about 80%) of vascular plant species occurs on at least two continents. An even larger proportion (90%) of bryophyte species occurs in both the North American and Eurasian Arctic.
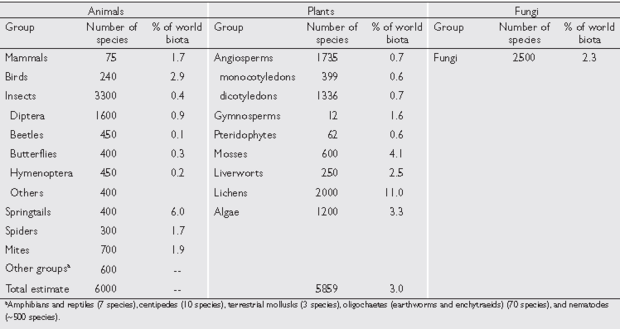
About 40% of arctic vascular plants (and a much higher percentage of mosses and lichens) are basically boreal species that now barely penetrate the Arctic (Table 7.3). They currently occur close to the treeline or along large rivers that connect the subarctic with the Arctic.These boreal species within the Arctic will probably be the primary boreal colonizers of the Arctic in the event of continued warming. Polyzonal (distributed in several zones), arctoboreal (in taiga and tundra zones), and hypoarctic (in the northern taiga and southern part of the tundra zone) species have even greater potential to widen their distribution and increase their abundance in a changing climate.The majority of cryptogams have wide distributions throughout the Arctic. Such species are likely to survive a changing climate, although their abundance is likely to be reduced (sections 7.3.3.1 and 7.4.1.2).
Table 7.3. Current diversity changes with latitude in the Arctic, excluding limnic and marine animals (compiled and modified from Matveyeva and Chernov.) General information about how species within the various categories are likely to respond to changes in climate and UV radiation levels is presented in the text, but insufficient information is available for most of the species in the table. | ||||
Category |
Optimum of distribution |
Examples | ||
Plants |
Birds |
Mammals and invertebrates | ||
Polyzonal |
Different zones in the Holarctic; within the tundra zone usually in local habitats and wet depressions |
Soil algae; the mosses Hylocomium splendens sensu lato, Aulacomnium turgidum, and Racomitrium lanuginosum; the liverwort Ptilidium ciliare; the lichens Cetraria islandica, Psora decipiens, and Cladina rangiferina; the vascular species Cardamine pratensis, Chrysosplenium alternifolium, and Eriophorum angustifolium; the sedge Carex duriuscula; the herb Helictotrichon krylovii; and the moss Tortula ruralis |
Common raven (Corvus corax), peregrine falcon (Falco peregrinus), white wagtail (Motacilla alba), northern wheatear (Oenanthe oenanthe) |
Wolf (Canis lupus), ermine (Mustela erminea), weasel (M. nivalis), voles (Microtus gregalis and M. oeconomus), and the mite Chiloxanthus pilosus |
Zonal boreal |
Not abundant and constrained to the southern Arctic in benign habitats such as river valleys, south-facing slopes, and wet areas |
Tree species of Larix; the orchid Corallorrhiza; the shrub Salix myrtilloides; the sedge Carex chordorrhiza; the herbs Allium schoenoprasum, Cortusa matthioli, Galium densiflorum, and Sanguisorba officianalis; and the forest mosses Climacium dendroides, Pleurozium shreberi, and Rhytidiadelphus triquetrus |
The forest birds Turdus iliacus and T. pilarisPhylloscopus borealis) and yellowbrowed warbler (P. inornatus); and the "river" ducks Anas acuta, A. penelope, and A. crecca (thrushes); the leaf warblers Arctic warbler ( |
Reindeer/caribou (Rangifer tarandus), wolverine (Gulo gulo), and brown bear (Ursus arctos) |
Zonal Arctic |
||||
Hypoarctic |
Optima in the southern tundra subzone |
The shrubs Betula nana and B. exilis and the sedge Eriophorum vaginatum |
Ptarmigan (Lagopus lagopus), spotted redshank (Tringa erythropus), little bunting (Emberiza pusilla), bar-tailed godwit (Limosa lapponica) |
The vole Microtus middendorffi, the ground beetle Carabus truncaticollis, the bumblebee Bombus cingulatus, and the spider Alopecosa hirtipes |
Hemiarctic |
Throughout the tundra zone but most frequent in the middle |
Most of the dominant species, including the grasses Arctophila fulva and Dupontia fisheri; the sedges Carex bigelowii/arctisibirica and C. stans; the shrub willow Salix reptans; the dwarf shrubs Dryas punctata/octopetala and Cassiope tetragona; the mosses Tomentypnum nitens, Drepanocladus intermedius, and Cinclidium arcticum; the herbs Lagotis minor and Pedicularis hirsuta; and the moss Polytrichum juniperinum |
Lapland longspur (Calcarius lapponicus), lesser golden plover (Pluvialis dominica), Pacific golden plover (P. fulva), and the dunlins Calidris alpina and C. minuta |
The lemming Lemmus sibiricus, the bumblebee Bombus balteatus, the ground beetles Curtonotus alpinus and Pterostichus costatus, and the flower-fly Parasyrphus tarsatus |
Euarctic |
Northern part of the tundra zone, rare in the southern part |
The dwarf shrubs Salix polaris and S. arctica |
Black-bellied plover (Pluvialis squatarola), curlew sandpiper (Calidris ferruginea), snowy owl (Nyctea scandiaca), snow bunting (Plectrophenax nivalis) and several more |
The lemming Dicrostonyx torquatus, the bumblebees Bombus hyperboreus and B. polaris, and the crane fly Tipula carinifrons |
Hyperarctic |
Polar desert and the northernmost part of the tundra zone |
Almost no plants are restricted to these zones; the following have their highest frequencies there: the grasses Phippsia algida and Poa abbreviata; the herbs Cerastium regelii, Draba oblongata, D. subcapitata, Saxifraga hyperborea, and S. oppositifolia; the mosses Dicranoweisia crispula, Bryum cyclophyllum, Orthothecium chryseon, and Seligeria polaris; and the lichens Cetrariella delisei, Arctocetraria nigricascens, Dactylina ramulosa, D. madreporiformis, and Thamnolia subuliformis |
The wader species Calidris alba and C. canutus |
No terrestrial mammal species are restricted to this zone. The collembolan Vertagopus brevicaudus |
a"steppe" species; bbizonal steppe and tundra species; cthis group characterizes the southern tundra subzone; dthis group is relatively small, but is valuable in subdividing the tundra zone into subzones. |
In contrast to the low diversity of the arctic flora at the continental and regional scales, individual communities (100 m2 plots) within the Arctic have a diversity similar to or higher than those of boreal and temperate zones. These diversities are highest in continental parts of the Arctic such as the Taymir Peninsula of Russia, where there are about 150 species of plants (vascular plants, lichens, and mosses) per 100 m2 plot, 40 to 50 species per square meter, and up to 25 species per square decimeter[5].
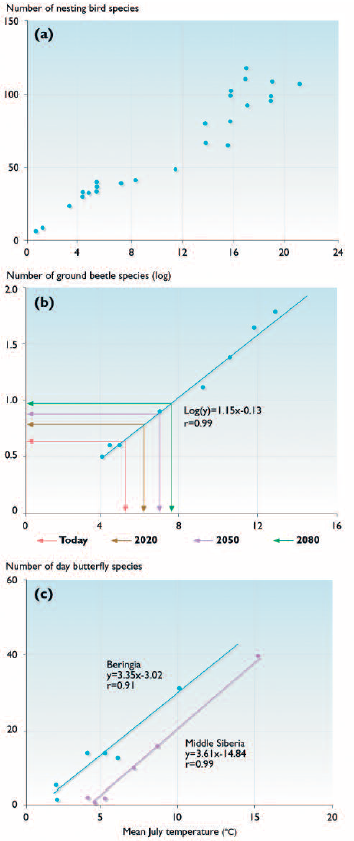
Latitudinal gradients of species diversity
Latitudinal gradients suggest that arctic plant diversity is sensitive to climate.The number of vascular plant species declines five-fold from south to north on the Taymir Peninsula[7]. Summer temperature is the environmental variable that best predicts plant diversity in the Arctic[8]. Other factors are also important, however: regions at different latitudes that have a similar maximum monthly temperature often differ in diversity. Taymir biodiversity values are intermediate between the higher values in Chukotka and Alaska, which have a more complicated relief, geology, and floristic history, and the lower values in the eastern Canadian Arctic with its impoverished flora resulting from relatively recent glaciation. All diversity values on the Yamal Peninsula are even lower than in Canada because of a wide distribution of sandy soils and perhaps its young age. Similar patterns are observed with butterflies (Fig. 7.5c) and spiders[9].
Therefore, latitudinal gradients of species diversity are best described as several parallel gradients, each of which depends on summer heat, but which may differ from one geographic region to another. This must be taken into consideration when projecting future changes in biodiversity. Figure 7.5b illustrates how current bioclimatic distributions are related to climate change scenarios by plotting the likely changes in the number of ground beetles for three time slices of mean July temperature derived from the mean of the scenarios generated by the five ACIA-designated models.
At the level of the local flora (the number of species present in a landscape of about 100 km2), there is either a linear or an “S”-shaped relationship between summer temperature and the number of species (Fig. 7.6). The number of species is least sensitive to temperature near the southern margin of the tundra and most sensitive to temperatures between 3 and 8 ºC. This suggests that the primary changes in species composition are very likely to occur in the northern part of the tundra zone and in the polar desert, where species are now most restricted in their distribution by summer warmth and growingseason length. July temperature, for example, accounts for 95% of the variance in number of vascular plant species in the Canadian Arctic[12], although extreme winter temperatures are also important (Section 7.3.3.1 (Implications of current species distributions for future biotic change in the Arctic)). Summer warmth, growing-season length, and winter temperatures all affect the growth, reproduction, and survival of arctic plants.The relative importance of each varies from species to species, site to site, and year to year.
The steep temperature gradient that has such a strong influence on species diversity occurs over much shorter distances in the Arctic than in other biomes. North of the treeline in Siberia, mean July temperature decreases from 12 to 2 ºC over 900 km, whereas mean July temperature decreases by 10 ºC over 2000 km in the boreal zone, and decreases by less than 10 ºC from the southern boreal zone to the equator[13].
Comparing the temperature decrease of 10 ºC with the projected 2.5 ºC increase in mean July temperature in the Arctic by 2080 (mean of the two extremes – 1.1 and 4.2 ºC – projected by the five ACIA-designated models) suggests that much of the Arctic is very likely to remain within the arctic summer climate envelope (although the increase in winter temperature is projected to be higher).
Because of the steep latitudinal temperature gradients in the Arctic, the distance that plants must migrate in response to a change in temperature is much less in the Arctic than in other biomes, particularly where topographic variations in microclimate enable plants to grow far north of their climatic optima. The low solar angle and presence of permafrost make topographic variations in microclimate and associated plant community composition particularly pronounced in the Arctic. Thus, both the sensitivity of arctic species diversity to temperature and the short distance over which this temperature gradient occurs suggest that arctic diversity is very likely to respond strongly and rapidly to high-latitude temperature change.
Latitudinal patterns of diversity differ strikingly among different groups of plants (Table 7.3). Many polyzonal, boreal, and hypoarctic species have ranges that extend into the Arctic. Some of these (e.g., the moss Hylocomium splendens and the sedges Eriophorum angustifolium and E. vaginatum) are important dominants within the Arctic. Tussocks of E. vaginatum structure the microtopography of broad areas of tussock tundra[14], and H. splendens exerts a control over nutrient cycling[15]. Tall willow (Salix spp.) and alder (Alnus fruticosa) shrubs as well as dwarf birch (Betula exilis and B. nana) form dense thickets in the southern part of the tundra zone and often have outlier populations that extend far to the north in favorable habitats[16].
Species that are important community dominants are likely to have a particularly rapid and strong effect on ecosystem processes where regional warming occurs. Hemiarctic species are those that occur throughout the Arctic. Many of these species are common community dominants, including Carex bigelowii/arctisibirica, C. stans, Dryas octopetala/punctata, Cassiope tetragona, and the moss Tomentypnum nitens. Due to their current widespread distribution, their initial responses to climatic warming are likely to be increased productivity and abundance, probably followed by northward extension of their ranges. The species most vulnerable to climate change are likely to be euarctic (polar willow – Salix polaris) and hyperarctic species that now have the greatest abundance and widest ecological amplitude in the northernmost part of the tundra zone and in polar deserts, respectively.These groups of species are best adapted to the climate conditions of the high Arctic where they are distributed in a wide range of habitats where more competitive southern species are absent. In the more southerly regions of the tundra zone, they are able to grow only (or mainly) in snow beds (depressions in the landscape where snow accumulates and plant growth and diversity is limited by particularly short snow-free periods). It is likely that their ecological amplitude will narrow and their abundance decrease during climate warming.
Thus, responses to climate change will be different in various groups of plants. Some currently rare boreal species are likely to move further north and the relative abundance and range of habitats occupied by more common species are likely to increase. When southern species with currently narrow niches penetrate into the harsher ecosystems at high latitudes, therefore, there is likely to be a broadening of their ecological niches there. In contrast, some true arctic species (endemics) that are widespread at high latitudes are likely to become more restricted in their local distribution within and among ecosystems.They could possibly disappear in the lower latitudes where the tundra biome is particularly narrow. Only few high-arctic plants in Greenland are expected to become extinct, for example, Ranunculus sabinei, which is limited to the narrow outer coastal zone of North Greenland[17]. However, temperature is not the only factor that currently prevents some species from being distributed in the Arctic. Latitude is important, as life cycles depend not only on temperature but on the light regime as well. It is very likely that arctic species will tolerate warmer summers whereas long day lengths will initially restrict the distribution of some boreal species ([[Section 7.3.3.1 (Implications of current species distributions for future biotic change in the Arctic)]2]). New communities with a peculiar species composition and structure are therefore very likely to arise, and will be different from those that exist at present.
Animals (7.3.1.2)
Species diversity
The diversity of arctic terrestrial animals beyond the latitudinal treeline (6000 species) is nearly twice as great as that of vascular plants and bryophytes[18] (Table 7.2). As with plants, the arctic fauna accounts for about 2% of the global total, and, in general, primitive groups (e.g., springtails, 6% of the global total) are better represented in the Arctic than are advanced groups such as beetles (0.1% of the global total[19]).There are about 322 species of vertebrates in the Arctic, including approximately 75 species of mammals, 240 species of birds, 2 species of reptiles, and 5 species of amphibians. Insects are the most diverse group of arctic animals; of approximately 3300 arctic species, about 50% are Diptera, 10% are beetles (Coleoptera), 10% are butterflies (Lepidoptera), and 10% are Hymenoptera. The Arctic has about 300 species of spiders (Arachnida), 700 species of mites (Acarina), 400 species of springtails (Collembola), 500 species of nematodes, 70 species of oligochaetes (of which most are Enchytraeidae), only a few mollusks, and an unknown number of protozoan species.
In the Arctic as defined by CAFF (Conservation of Arctic Flora and Fauna) (which includes forested areas), some 450 species of birds have been recorded breeding. Some species extend their more southern breeding areas only marginally into the Arctic. Others are not migratory and stay in the Arctic throughout the year. About 280 species have their main breeding distribution in the Arctic and migrate regularly[20]. An estimate of the total number of individuals involved is not possible, as too little is known about the population size of most species or their arctic proportion. However, a rough first approximation suggests that there are at least several hundred million birds. Population sizes of water birds are better known and the Arctic is of particular importance for most water birds, such as divers, geese, and waders. Twelve goose species breed in the Arctic, eleven almost entirely and eight exclusively.These comprise about 8.3 million birds.The total number of arctic-breeding sandpipers (24 species) exceeds 17.5 million birds[21]. The total number of water birds, including other wader species, divers, swans, ducks, and gulls is estimated to be between 85 and 100 million birds.
Latitudinal gradients of species diversity
Latitudinal patterns of diversity in arctic animals are similar to those described for arctic plants. Species diversity declines in parallel with decreasing temperature in most animal groups (Fig. 7.5), including birds, ground beetles, and butterflies[22]. However, in some groups (e.g., peatland birds and sawflies at local sites in the European Arctic), concentrations of both species diversity and density per unit area can increase compared with more southern territories, perhaps because the habitat types appropriate to these groups are more diverse in the tundra than in the boreal forest. In general, the latitudinal decline in the number of animal species is more pronounced (frequently greater than 2.5-fold) than in vascular plants. As with plants, at a given temperature there are more animal species in Beringia, with its complicated relief, geology, and biogeographic history than in the Taymir Peninsula. Many animal species are restricted to the boreal zone because they depend on the crown, wood, roots, or litter of trees, which are absent in the tundra zone. These groups include wood-boring insects and wooddecaying fungi and their predators[23], as well as mammals and birds that specialize on tree seeds and leaves. Other important animals, including the raven (Corvus corax), wolf, red fox (Vulpes vulpes), and ermine (Mustela erminea), are primarily boreal in distribution but remain an important component of many arctic ecosystems.There are a few terrestrial animals restricted to the high Arctic such as the sanderling (Calidris alba) and a common Collembola, Vertagopus brevicaudus. Other arctic species have their centers of distribution in the northern, mid- or southern Arctic (Table 7.3).The more diverse patterns of animal distribution compared to plants make it more difficult to project how animals will respond to climatic warming. Some herbivores have distributions that are more limited than those of their host plants[24], so it is possible that warming will allow these species to extend northward relatively rapidly.
As with plants, latitudinal patterns of diversity differ strikingly among different groups of animals (Table 7.3).The common species tend to be more broadly distributed in the far north. In northern Taymir, there are only 12 species of springtails but 80% of these occur in all microsites and topographic locations investigated[25]. Some boreal birds, such as the American robin (Turdus migratorius), penetrate only into the southern part of the tundra while others can occur far from the area of their continuous distribution (climatic optimum): in the vicinity of Dixon (Taymir), the forest thrushes T. pilaris and T. iliacus form populations in the northernmost part of the tundra zone, 400 km from the last outposts of the forests. At the southern limits of the tundra, there is greater specialization among microhabitats. Many more species occur in intrazonal habitats, occupying relatively small and isolated sites, than in zonal habitats that contain only a small proportion of the regional fauna. Warming is therefore likely to lead to more pronounced habitat and niche specialization.
An important consequence of the decline in numbers of species with increasing latitude is an increase in dominance. For example, one species of collembolan, Folsomia regularis, may constitute 60% of the total collembolan density in the polar desert[26].These “super-dominant” species are generally highly plastic, occupy a wide range of habitats, and generally have large effects on ecosystem processes. Lemmings (Lemmus spp. and Dicrostonyx spp.) are superdominant species during peak years of their population cycles[27] and have large effects on ecosystem processes[28].
Microorganisms (7.3.1.3)
Species diversity
Microbial organisms are critically important for the functioning of ecosystems, but are difficult to study and are poorly understood compared with other species. However, the International Biological Programme[29] significantly advanced understanding of arctic microorganisms, compared with those of other biomes, when an inventory of microbial communities was undertaken in the tundra[30]. At the beginning of the 21st century, the knowledge of microbial diversity in the tundra remains little better than 30 to 40 years ago, and recent outstanding progress in molecular microbial ecology has rarely been applied to arctic terrestrial studies.
Presently there are 5000 to 6000 named bacterial species globally and about the same number of fungi[31], compared with more than one million named plant and animal species[32]. Some scientists have interpreted this difference to mean that the bacteria are not particularly diverse[33]. However, there are several reasons (Section 7.7.1.1 (Implications of current species distributions for future biotic change in the Arctic)) to believe that the apparent limited diversity of microbes is an artifact.
Recent progress in molecular biology and genetics has revolutionized bacterial classification and the understanding of microbial phylogeny (“family trees”) and biodiversity in general.The DNA sequencing technique has reorganized bacterial classification and brought order to microbial taxonomy[34]. Moreover, the microbial inventory can now be done without isolation and cultivation of the dominant microorganisms, because it is enough to extract the total community DNA from the soil and amplify, clone, and sequence the individual genes.This culture-independent approach has been applied occasionally for analysis of microbial communities in subarctic and arctic soils, most often to study relatively simple communities in hot springs, subsoils, and contaminated aquifers (Section 7.3.5.2 (Implications of current species distributions for future biotic change in the Arctic)). Analysis of Siberian subsurface permafrost samples[35] resulted in the formation of a clone library of 150 clones which has been separated into three main groups of Eubacteria. From 150 clones so far analyzed, the authors have identified several known species (Arthrobacter, Clostridium, and Pseudomonas), while the most abundant phylotypes were represented by completely unknown species closely affiliated with iron-oxidizing bacteria.
Another area of intensive application of molecular tools was northern wetlands (cold, oligotrophic (nutrient poor), and usually acidic habitats) related to the methane cycle (sections 7.4 and 7.5).The most challenging tasks were to determine what particular microbial organisms are responsible for the generation and uptake of methane (so-called methanogens and methanotrophs) in these northern ecosystems and what their reaction might be to warming of arctic soils. It was found that most of the boreal and subarctic wetlands contain a wide diversity of methanogens[36] and methanotrophs[37], most of them distantly related to known species. Only recently, some of these obscure microbes were obtained in pure culture or stable consortia[38]. The novel microbes of methane cycles are extreme oligotrophic species that evolved to function in media with very low concentrations of mineral nutrients.Taxonomically, these methanogens form new species, genera, and even families within the Archaea domain[39]. The acidophilic methanotrophs form two new genera: Methylocapsaand Methylocella[40], the latter affiliating with heterotrophic Beijerinckia indica.
A DNA-based technique allows determination of the upper limit for variation of microbial diversity in the Arctic as compared with other natural ecosystems, that is, how many species (both cultured and unculturable) soils contain.This technique is called DNA reassociation (how quickly the hybrid double helix is formed from denatured single-stranded DNA).
Arctic polar desert and tundra contain considerable microbial diversity, comparable with boreal forest soils and much higher than arable soils. Although extreme environmental conditions restrain the metabolic activity of arctic microbes, they preserve huge potential that is likely to display the same activity as boreal analogues during climate warming.
There is a much higher degree of genomic diversity in prokaryotic communities (prokaryotes such as cyanobacteria have a simple arrangement of genetic material whereas eukaryotes such as microalgae have genetic material arranged in a more advanced way, in that the DNA is linear and forms a number of distinct chromosomes) of heterogeneous habitats (virgin soils, pristine sediments) as compared with more homogeneous samples: the DNA diversity found in 30 to 100 cc of heterogeneous samples corresponds to about 104 different genomes, while in pond water and arable soils the number of genomes decreases to 100 to 102. Based on extrapolation and taking into account that listings of species can significantly overlap for microbial communities of different soils, a rough estimate is that there could be from 104 to 109 prokaryotic species globally[41].
The conventional inventory approach based on cultivation suggests that at present in the Arctic, it is possible to identify in any particular soil no more than 100 prokaryotic species from the potential of 1000 to 3000 “genome equivalents” (Table 7.4) and no more than 2000 species of eukaryotes. In the broadly defined Russian Arctic, 1750 named fungi species (not including yeast and soil fungi) have been identified[44]. About 350 of these are macromycetes. However, the number of fungi species in the Arctic proper is 20 to 30% less, but these data are far from complete.The Arctic has fewer species of bacteria, fungi, and algae than other major biomes; actinomycetes are rare or absent in most tundra sites[42]. While most major phyla of microflora are represented in tundra ecosystems, many species and genera that are common elsewhere, even in subarctic ecosystems, are rare or absent in tundra. Gram-positive bacteria, including gram-positive spore forms, are absent or rare in most tundra sites. Arthrobacter and Bacillus can rarely be isolated and then only from drier areas. Azotobacter, the freeliving nitrogen-fixing bacterium, is extremely rare in tundra, and the moderate rate of nitrogen fixation observed in situ is mainly due to the activity of cyanobacteria. Sulfur-oxidizing bacteria are also reported to be rare or absent. Even using enrichment techniques, Bunnell et al.[43] rarely found chemoautotrophic sulfur-oxidizing bacteria. Photosynthetic sulfur bacteria were not found in any IBP tundra biome sites and were reported in only one subarctic site[44], although they are common in coastal areas of the west and south coasts of Hudson Bay. Sulfur-reducing bacteria, while not abundant in tundra sites, have been reported in sites in the Arctic and Antarctic. Iron-oxidizing bacteria are very rare in tundra sites. Despite ample iron substrate in tundra ponds and soils, chemoautotrophic ferrous iron oxidizers were not found in IBP tundra sites[45]. In contrast, methanotrophic and methanogenic bacteria appear to be widespread in tundra areas.
As with bacteria, many generally common fungi are conspicuous by their rare occurrence or absence in tundra areas. Aspergillus, Alternaria, Botrytis, Fusarium, and Rhizopus simply do not occur and even Penicillium are rare[46]. Yeasts can be isolated readily but there is very low species diversity in culture media. Only three different species were reported for Point Barrow tundra[47]. Aquatic fungi show high diversity, especially Chytridiales and Saprolegniales. However they may not be endemic, and reflect the annual migration into the Arctic of many avian species, especially waterfowl.The so-called higher fungi, Basidiomycetes and Ascomycetes, also have low diversity.They are reduced in the Arctic to 17 families, 30 genera, and about 100 species. In comparison, subarctic and temperate regions contain at least 50 families, not less than 300 genera, and anywhere up to 1200 species[48]. Mycorrhizal symbionts on tundra plants are common. Arbuscular, ecto-, ericoid, arbutoid, and orchid mycorrhizal fungi are associated with plants in arctic ecosystems[49]. The ectomycorrhizal symbionts are important as they form mycorrhizal associations with Betula, Larix, Pinus, Salix, Dryas, Cassiope, Polygonum, and Kobresia. Based on fungal fruitbodies, Borgen et al.[50] estimate that there are 238 ectomycorrhizal fungal species in Greenland, which may increase to around 250 out of a total of 855 basidiomyceteous fungi in Greenland when some large fungal genera such as Cortinarius and Inocybe have been revised.With the exception of Eriophorum spp., Flanagan[51] found endotrophic Arbuscula-like mycorrhizae on all ten graminoid plants examined.The number of fungal species involved in other mycorrhizal symbioses is not clear.
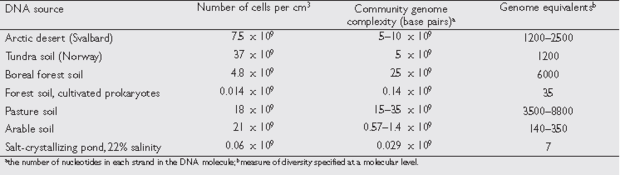
Tundra algae exhibit the same degree of reduction in species diversity seen among the fungi and bacteria[53], which documents a diversity much reduced from that of the microflora of temperate regions. Cyanobacteria and microalgae are among the oldest (in evolutionary terms) and simplest forms of life on the planet that can photosynthesize. Unicellular and filamentous photosynthetic cyanobacteria and microalgae are among the main primary colonizers adapted to conditions of the arctic terrestrial environment.They are widespread in all terrestrial and shallow wetland habitats and frequently produce visible biomass.Terrestrial photosynthetic microorganisms colonize mainly the surface and subsurface of the soil and create the crust[54]. Algal communities in shallow flowing or static wetlands produce mats or mucilaginous clusters that float in the water but are attached to rocks underneath[55]. Terrestrial and wetland habitats represent a unique mosaic of cyanobacteria and algae communities that occur up to the highest and lowest possible latitudes and altitudes as long as water (liquid or vapor) is available for some time during the year[56].The arctic soil and wetland microflora is composed mainly of species from Cyanobacteria, Chrysophyceae, Xanthophyceae, Bacillariophyceae, Chlorophyceae, Charophyceae, Ulvophyceae, and Zygnemaphyceae. A wide range of species diversity (between 53 and 150 to 160 species) has been reported from various arctic sites[57].
Latitudinal gradients of species diversity
Arctic soils contain large reserves (standing crops) of microbial (mainly fungal) biomass, although the rate of microbial growth is generally lower than in the boreal zone. Surprisingly, under severe arctic conditions, soil microbes fail to produce spores and other dormant structures that are adaptations to harsh environments (Fig. 7.7).The species diversity of all groups of soil microorganisms is lower in the Arctic than further south, decreasing from about 90 species in Irish grassland, through about 50 species in Alaskan birch forest, to about 30 species in Alaskan tundra[58]. As with plants and animals, there are large reductions in numbers of microbial species with increasing latitude, although these patterns are less well documented. A correlate of the decreasing number of species with increasing latitude is increasing dominance of the species that occur, as with plants and animals. One yeast (Cryptococcus laurentii), for example, constitutes a large proportion of yeast biomass across a range of community types in the northern Taymir Peninsula[59].
The hyphal length of fungi in the Arctic shows a latitudinal trend in which the abundance of fungi, as measured by hyphal length, decreases toward the north. Although it is not known if this trend also applies to the species diversity of fungal mycelia (the belowground network of fungal filaments or hyphae), it is clear that the amount of fungal hyphae is low in the Arctic[60]. In the high Arctic, fungal hyphal length was 23±1 m/g in a polar semi desert on Svalbard (78º56' N), 39 m/g on a beach ridge, and 2228 m/g in a mesic meadow on Devon Island (75º33' N). At Barrow, Alaska, hyphal length was 200 m/g. In a subarctic mire in Swedish Lapland, hyphal length was 3033 m/g.These values can be compared with 6050 to 9000 m/g in temperate uplands in the United Kingdom and 1900 to 4432 m/g in temperate woodland soils.
Summary (7.3.1.4)
Species diversity appears to be low in the Arctic, and decreases from the boreal forests to the polar deserts of the extreme north. Only about 3% (~5900 species) of the global flora (excluding algae) occur in the Arctic north of the treeline. However, primitive plant species (mosses and lichens) are particularly abundant. Although the number of plant species in the Arctic is low in general, individual communities of small arctic plants have a diversity similar to or higher than those of boreal and temperate zones: there can be up to 25 species per square decimeter. Latitudinal gradients suggest that arctic plant diversity is sensitive to climate, and species number is least sensitive to temperature near the southern margin of the tundra.The temperature gradient that has such a strong influence on species diversity occurs over much shorter distances in the Arctic than in other biomes. The diversity of arctic animals beyond the latitudinal treeline (~6000 species) is nearly twice as great as that of vascular plants and bryophytes. As with plants, the arctic fauna accounts for about 3% of the global total, and in general, primitive groups (e.g., springtails) are better represented in the Arctic than are advanced groups such as beetles. In general, the decline in animal species with increasing latitude is more pronounced than that of plants (frequently greater than 2.5-fold). An important consequence of the decline in numbers of species with increasing latitude is an increase in dominance. “Super-dominant” plant and animal species occupy a wide range of habitats, and generally have large effects on ecosystem processes.
Microbial organisms are more difficult to enumerate. Arctic soils contain large reserves of microbial biomass, although diversity of all groups of soil microorganisms is lower in the Arctic than further south. Many common bacteria and fungi are rare or absent in tundra areas. As with plants and animals, there are large reductions in numbers of microbial species with increasing latitude, and increasing dominance of the species that occur. The latitudinal temperature gradient within tundra is steeper than for any other biome, and outlier populations of more southerly species frequently exist in favorable microenvironments far to the north of their centers of distribution. Consequently, migration of southerly taxa is very likely to occur more rapidly in the Arctic than in other biomes.Temperature-induced biotic change is likely to be most pronounced at the northern extreme of tundra, where species distributions are most temperature-sensitive.
The initial response of diversity to warming is likely to be an increase in the diversity of plants, animals, and microbes, and reduced dominance of species that are currently widespread.Taxa most likely to expand into tundra are boreal taxa that currently exist in river valleys and could spread into the uplands, or animal groups such as wood-boring beetles that are presently excluded due to a lack of food resources. Although current extreme environmental conditions restrain the metabolic activity of arctic microbes, they preserve huge potential that is ready to display the same activity as boreal analogues during climate warming.Warming could possibly cause the extinction of a few arctic plants that currently occur in narrow latitudinal strips of tundra adjacent to the sea. Some animals are arctic specialists and could possibly face extinction.Those plant and animal species that have their centers of distribution in the high or middle Arctic are most likely to show reduced abundance in their current locations should projected warming occur.
Chapter 7: Arctic Tundra and Polar Desert Ecosystems
7.1 Introduction (Implications of current species distributions for future biotic change in the Arctic)
7.2 Late-Quaternary changes in arctic terrestrial ecosystems, climate, and ultraviolet radiation levels
7.3 Species responses to changes in climate and ultraviolet-B radiation in the Arctic
7.3.1 Implications of current species distributions for future biotic change
7.3.2 General characteristics of arctic species and their adaptations in the context of changes in climate and ultraviolet-B radiation levels
7.3.3 Phenotypic responses of arctic species to changes in climate and ultraviolet-B radiation
7.3.4 Genetic responses of arctic species to changes in climate and ultraviolet-B radiation levels
7.3.5 Recent and projected changes in arctic species distributions and potential ranges
7.4 Effects of changes in climate and UV radiation levels on structure and function of arctic ecosystems in the short and long term
7.4.1 Ecosystem structure
7.4.2 Ecosystem function
7.5 Effects of climate change on landscape and regional processes and feedbacks to the climate system
7.6 Synthesis: Scenarios of projected changes in the four ACIA regions for 2020, 2050, and 2080
7.7 Uncertainties and recommendations
References
- {{note|40}Karatygin, I.V., E.L. Nezdoiminogo,Y.K. Novozhilov and M.P. Zhurbenko, 1999. Russian Arctic Fungi: A Checklist. St. Petersburg. 212pp.}
Citation
Committee, I. (2012). Implications of current species distributions for future biotic change in the Arctic. Retrieved from http://editors.eol.org/eoearth/wiki/Implications_of_current_species_distributions_for_future_biotic_change_in_the_Arctic- ↑ Matveyeva, N. and Y. Chernov, 2000. Biodiversity of terrestrial ecosystems. In: M. Nuttall and T.V. Callaghan (eds.). The Arctic:Environment, People, Policy, pp. 233–274. Harwood Academic Publishers.;-- Sekretareva, N.A., 1999.The Vascular Plants of the Russian Arctic and Adjacent Territories. Sofia, Moscow, 160pp.
- ↑ Murray, D.F., 1995. Causes of Arctic plant diversity: origin and evolution. In: F.S. Chapin III and C. Körner (eds.). Arctic and AlpineBiodiversity: Patterns, Causes and Ecosystem Consequences. Ecological Studies, 113:21–32.
- ↑ Afonina, O.M. and I.V. Czernyadjeva, 1995. Mosses of the Russian Arctic: check-list and bibliography. Arctoa, 5:99–142.;-- Andreev, M.P.,Yu.V. Kotlov and I.I. Makarova, 1996. Checklist of lichens and lichenicolous fungi of the Russian Arctic.The Bryologist, 99(2):137–169.;-- Konstantinova, N.A. and A.D. Potemkin, 1996. Liverworts of the Russian Arctic: an annotated check-list and bibliography. Arctoa, 6:125–150.
- ↑ Chernov,Y.I., 2002. Arctic biota: taxonomic diversity. Zoologicheski Zhurnal, 81(12):1411–1431. (In Russian with English summary);-- Matveyeva and Chernov, 2000, Op. cit.
- ↑ Matveyeva, N.V., 1998. Zonation in Plant Cover of the Arctic. Proceedings of the Komarov Botanical Institute, 21. Russian Academy of Sciences, Moscow, 219pp. (In Russian)
- ↑ Chernov,Y.I., 1989. Heat conditions and Arctic biota. Ekologiya, 2:49–57. (In Russian)-- Chernov,Y.I., 1995. Diversity of the Arctic terrestrial fauna. In: F.S. Chapin III and C. Körner (eds.). Arctic and Alpine Biodiversity: Patterns, Causes and Ecosystem Consequences. Ecological Studies, 113:81–95.-- Matveyeva and Chernov, 2000, Op. cit
- ↑ Matveyeva, 1998, Op. cit.
- ↑ Young, S.B., 1971.The vascular flora of St. Lawrence Island with special reference to floristic zonation in the Arctic regions. Contributions from the Gray Herbarium, 201:11–115.
- ↑ Chernov,Y.I., 1989, 1995 Op. cit.
- ↑ Matveyeva and Chernov, 2000, Op. cit.
- ↑ Rannie,W.F., 1986. Summer air temperature and number of vascular species in Arctic Canada. Arctic, 39:133–137.
- ↑ Ibid.
- ↑ Chernov, 1995, Op. cit.
- ↑ Bliss, L.C. and N.V. Matveyeva, 1992. Circumpolar Arctic vegetation. In: F.S. Chapin III, R.L. Jefferies, J.F. Reynolds, G.R. Shaver, and J. Svoboda (eds.). Arctic Ecosystems in a Changing Climate: An Ecophysiological Perspective, pp. 59–89. Academic Press.
- ↑ Hobbie, S.E., 1996.Temperature and plant species control over litter decomposition in Alaskan tundra. Ecological Monographs, 66:503–522.
- ↑ Matveyeva and Chernov, 2000, Op. cit.
- ↑ Heide-Jørgensen, H.S. and I. Johnsen, 1998. Ecosystem vulnerability to climate change in Greenland and the Faroe Islands. Miljønyt, 33:1–266.
- ↑ Chernov, 1995, 2002, Op. cit.
- ↑ Chernov, 1995, Op. cit.-- Matveyeva and Chernov, 2000 Op. cit.
- ↑ Scott, D.A., 1998. Global Overview of the Conservation of Migratory Arctic Breeding Birds outside the Arctic. CAFF Technical Report 4. Conservation of Arctic Flora and Fauna, 134pp.
- ↑ Zöckler, C., 1998. Patterns in Biodiversity in Arctic Birds. WCMC Biodiversity Bulletin 3.World Conservation Monitoring Centre, Cambridge, 15pp.
- ↑ Chernov, 1995, Op. cit.
- ↑ Chernov,Y.I. and N.V. Matveyeva, 1997. Arctic ecosystems in Russia. In: F.E.Wielgolaski (ed.). Polar and Alpine Tundra. Ecosystems of the World, 3:361–507. Elsevier.
- ↑ Strathdee, A.T. and J.S. Bale, 1998. Life on the edge: insect ecology in Arctic environments. Annual Review of Entomology, 43:85–106.
- ↑ Chernov and Matveyeva, 1997, Op. cit.
- ↑ Babenko, A.B. and V.I. Bulavintsev, 1997. Springtails (Collembola) of Eurasian polar deserts. Russian Journal of Zoology, 1(2):177–184.
- ↑ Stenseth, N.C. and R.A. Ims, 1993.The Biology of Lemmings. Academic Press, UK, 683pp.
- ↑ Batzli, G.O., R.G.White, S.F. MacLean Jr., F.A. Pitelka and B.D Collier, 1980.The herbivore-based trophic system. In: J. Brown, P.C. Miller, L.L.Tieszen and F.L. Bunnell (eds.). An Arctic Ecosystem:The Coastal Tundra at Barrow, Alaska, pp. 335–410. Dowden, Hutchinson and Ross, Pennsylvania.;-- Batzli, G.O., R.G.White, S.F. MacLean Jr., F.A. Pitelka and B.D. Collier, 1980.The herbivore-based trophic system. In: J. Brown, P.C. Miller, L.L.Tieszen and F.L. Bunnell (eds.). An Arctic Ecosystem:The Coastal Tundra at Barrow, Alaska, pp. 335–410. Dowden, Hutchinson and Ross, Pennsylvania.;-- Stenseth and Ims, 1993, Op. cit.
- ↑ (IBP; 1964–1974)
- ↑ Heal, O.W., P.W. Flanagan, D.D. French and S.F. MacLean Jr., 1981. Decomposition and accumulation of organic matter in tundra. In: L.C. Bliss, O.W. Heal and J.J. Moore (eds.).Tundra Ecosystems: A Comparative Analysis, pp. 587–634. Cambridge University Press.
- ↑ Holt, J.G., N.R. Krieg, P.H.A. Sneath, J.T. Staley and S.T.Williams (eds.), 1994. Bergey’s Manual of Determinative Bacteriology, 9th Edition.Williams and Wilkins, 787pp.
- ↑ Mayr, E., 1998.Two empires or three? Proceedings of the National Academy of Sciences, 95:9720–9723.;-- Wilson, E.O., 1992.The Diversity of Life. Belknap Press
- ↑ Mayr, 1998, Op. cit.
- ↑ Wayne, L.G., D.J. Brenner, R.R. Colwell, P.A.D. Grimont, O. Kandler, M.I. Krichevsky, L.H. Moore,W.E.C. Moore, R.G.E. Murray, E. Stackebrandt, M.P. Starr and H.G.Truper, 1987. Report of the ad hoc committee on reconcilation of approaches to bacterial systematics. International Journal of Systematic Bacteriology, 37(4):463–464.}
- ↑ Gilichinsky, D., 2002. Permafrost as a microbial habitat. In: G. Bitton (ed.). Encyclopedia of Environmental Microbiology,Vol 6, pp. 932–956.Wiley.;-- Tsapin, A.I., G.D. McDonald, M.Andrews, R. Bhartia, S. Douglas and D. Gilichinsky, 1999. Microorganisms from permafrost viable and detectable by 16SRNA analysis: a model for Mars.The Fifth International Conference on Mars, July 19–23, 1999, Pasadena, California. Abstract 6104.
- ↑ Hales, B.A., C. Edwards, D.A. Ritchie, G. Hall, R.W. Pickup and J.R. Saunders, 1996. Isolation and identification of methanogen-specific DNA from blanket bog peat by PCR amplification and sequence analysis. Applied and Environmental Microbiology, 62:668–675.;-- Galand, P.E., S. Saarnio, H. Fritze and K.Yrjala, 2002. Depth related diversity of methanogen Archaea in Finnish oligotrophic fen. FEMS Microbiology Ecology, 42:441–449.
- ↑ McDonald, I.R., M. Upton, G. Hall, R.W. Pickup, C. Edwards, J.R. Saunders, D.A. Ritchie and J.C. Murrel, 1999. Molecular ecological analysis of methanogens and methanotrophs in blanket bog peat. Microbial Ecology, 38:225–233.-- Radajewsky, S., P. Ineson, N.R. Parekh and J.C. Murrell, 2000. Stableisotope probing as a tool in microbial ecology. Nature, 403:646–649.
- ↑ Dedysh, S.N., N.S. Panikov,W. Liesack, R. Grosskopf, J. Zhou and J.M.Tiedje, 1998. Isolation of acidophilic methane-oxidizing bacteria from northern peat wetlands. Science, 282:281–284.;-- Sizova, M.V., N.S. Panikov,T.P.Tourova and P.W. Flanagan, 2003. Isolation and characterization of oligotrophic acido-tolerant methanogenic consortia from a Sphagnum peat bog. FEMS Microbiology Ecology, 45:301–315.
- ↑ Sizova et al., 2003, Op. cit.
- ↑ (Dedysh et al., 1998, Op. cit.;-- Dedysh, S.N.,W. Liesack,V.N. Khmelenina, N.E. Suzina,Y.A.Trotsenko, J.D. Semrau, A.M. Bares, N.S. Panikov and J.M.Tiedje, 2000. Methylocella palustris gen. nov., sp. nov., a new methane-oxidizing acidophilic bacterium from peat bogs, representing a novel subtype of serine-pathway methanotrophs. International Journal of Systematic and Evolutionary Microbiology, 50:955–969.
- ↑ Staley, J.T. and J.J. Gosink, 1999. Poles apart: biodiversity and biogeography of sea ice bacteria. Annual Review of Microbiology, 53:189–215.;-- Torsvik,V., L. Øvreås and T. Frede Thingstad, 2002. Prokaryotic diversity – magnitude, dynamics, and controlling factors. Science, 296:1064–1066.
- ↑ Bunnell, F., O.K. Miller, P.W. Flanagan and R.E. Benoit, 1980.The microflora: composition, biomass and environmental relations. In: J. Brown, P.C. Miller, L.L.Tieszen and F.L. Bunnell (eds.). An Arctic Ecosystem:The Coastal Tundra at Barrow, Alaska, pp. 255–290. Dowden, Hutchinson and Ross, Pennsylvania..
- ↑ Ibid.
- ↑ Ibid.;-- Dunican, L.K. and T. Rosswall, 1974 Taxonomy and physiology of tundra bacteria in relationship to site characteristics. In: A.J. Holding, O.W. Heal, S.F. MacLean Jr. and P.W. Flanagan (eds.). Soil Organisms and Decomposition in Tundra, pp. 79–92. International Biological Programme Tundra Biome Steering Committee, Stockholm.
- ↑ Ibid.
- ↑ Flanagan, P.W. and W. Scarborough, 1974. Physiological groups of decomposer fungi in tundra plant remains. In: A.J. Holding, O.W. Heal, S.F. MacLean Jr. and P.W. Flanagan (eds.). Soil Organisms and Decomposition in Tundra, pp. 159–181. International Biological Programme Tundra Biome Steering Committee, Stockholm.
- ↑ Bunnell et al., 1980, Op. cit.
- ↑ Miller, O.K. and D.F. Farr, 1975. Index of the Common Fungi of North America, Synonymy and Common Names. Bibliotheca Mycologia, 44:206–230. J. Cramer,Vaduz.
- ↑ Michelsen, A., C. Quarmby, D. Sleep and S. Jonasson, 1998.Vascular plant 15N abundance in heath and forest tundra ecosystems is closely correlated with presence and type of mycorrhizal fungi in roots. Oecologia, 115:406–418.
- ↑ Borgen et al. (in press)
- ↑ Flanagan (unpub. data, 2004)
- ↑ After Torsvik et al., 2002, Op. cit.
- ↑ Bunnell et al., 1980, Op. cit.;-- Elster, J., J. Svoboda, S. Ohtani and H. Kanda, 2002. Feasibility studies on future phycological research in polar regions. Polar Bioscience, 15:114–122.;-- Fogg, G.E., 1998.The Biology of Polar Habitats. Oxford University Press, 274pp.
- ↑ Elster, J., A. Lukesova, J. Svoboda, J. Kopecky and H. Kanda, 1999. Diversity and abundance of soil algae in the polar desert, Sverdrup Pass, central Ellesmere Island. Polar Record, 35(194):231–254.
- ↑ Elster, J., J. Svoboda, J. Komárek and P. Marvan, 1997. Algal and cyanoprocaryote communities in a glacial stream, Sverdrup Pass, 79º N, Central Ellesmere Island, Canada. Archiv fuer Hydrobiologie. Supplementband. Algological Studies, 85:57–93.
- ↑ Elster, J., J. Svoboda, S. Ohtani and H. Kanda, 2002. Feasibility studies on future phycological research in polar regions. Polar Bioscience, 15:114–122.
- ↑ Ibid.
- ↑ Flanagan and Scarborough, 1974, Op. cit.
- ↑ Chernov,Y.I., 1985. Synecological analysis of yeasts in the Taimyr tundra. Ekologiya, 1:54–60.
- ↑ Robinson, C.H., O.B. Borisova,T.V. Callaghan and J.A. Lee, 1996. Fungal hyphal length in litter of Dryas octopetala in a high-Arctic polar semi-desert, Svalbard. Polar Biology, 16:71–74.
- ↑ (recalculated from data in Mirchink, 1988)