Metal hydrides for hydrogen fuel
Metal hydrides are simple chemical compounds that form when metals react with hydrogen gas. In general, the reaction for the formation of metal hydrides can be written as:
One can envision the reaction by considering a metal to be a lattice structure of individual atoms with small spaces in between. The hydrogen gas will then split to form two hydride ions. The hydride ion is very small, consisting of only a proton and an electron, and can fit into the small spaces in the metal lattice structure. The hydride ion (H-) is distinctly different from the hydrogen ion (H+). The hydrogen ion is formed when the hydrogen atom loses its electron whereas the hydride ion forms when diatomic hydrogen gas is cleaved.
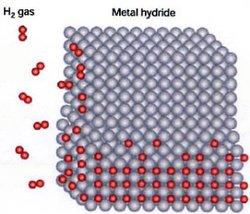
Hydrogen gas is currently used in industrial processes such as hydrogenation and the creation of chemicals such as ammonia, methanol, and acids. More recently, hydrogen gas has become an alternative energy source. There are multiple options for storing the hydrogen gas but no one method has proven to be the best.
Hydrogen gas is not an energy source, but an energy carrier. Even though water would be the only exhaust from a hydrogen fuel vehicle, the primary energy source may have produced greenhouse gases or air pollutants. There are many methods to produce hydrogen gas including steam/natural gas reformation, coal gasification, nuclear energy, and renewable sources such as wind, solar, and hydro power. Currently, each of these methods are limited by either their environmental impacts, prohibitive costs, or restrictive scale. An appropriate analysis of the environmental issues of using hydrogen as a fuel versus the fuels currently used necessitates a big picture approach considering both the energy carrier and the primary energy source.
Metal hydrides may be a viable storage alternative for hydrogen gas. There are many challenges ahead for developing metal hydrides, but both the US Department of Energy and the International Energy Agency have commissioned multiple metal hydride projects in hopes of creating a practical storage medium. The current methods of hydrogen storage, future uses of metal hydrides, challenges of widespread use, and some specific metal hydride chemistry are discussed in detail below.
Contents
Current Methods of Hydrogen Storage
Gaseous Hydrogen
Gaseous hydrogen is currently the most common method of hydrogen storage. The major problem with this is that hydrogen is a low density gas, meaning that it takes up a large amount of space. To counter this problem, it needs to be stored under high pressures, making it hard to transport and somewhat dangerous due to its explosive nature. This low density also makes it impractical for use in vehicular transportation as only a small amount of the gas could ever be stored in the fuel tank.
Liquid Hydrogen
Liquid hydrogen is often used as a storage medium because it increases the gravimetric density of the fuel and it is easier to transport over long distances than gaseous hydrogen. The main problem with liquefied hydrogen is that it must be stored at high pressures and incredibly low temperatures. To remain in the liquid phase, hydrogen must be pressurized and stored at −423.17 °F (−252.87°C). Additional energy is required in order to pressurize and cool the hydrogen to this point, negating the energy stored in the hydrogen itself.
Metal Hydride Chemistry
Metal hydrides are able to undergo a very simple chemical reaction with water to liberate the hydrogen bonded to the metal. This simple chemistry is the main reason why metal hydrides are considered to be an important hydrogen storage technology. The general equation for the liberation of hydrogen can be viewed as:
MH(s) + H2O(l) → H2 (g) +MOH
The metal hydride (usually a powdery solid) reacts with water to produce hydrogen gas and a hydroxide byproduct. Though this simple reaction appears promising on paper, there are still practical limitations to overcome before metal hydride chemistry is used in any main stream applications.
A large amount of metal hydride research also focuses on hydriding and dehydriding the metal without using the reaction with water noted above. This is accomplished by applying high pressure hydrogen to the metal for the hydriding process and adding a significant amount of energy for the dehydriding process. Many of the pure metals (Mg for example) are unstable at room temperature and react vigorously with water vapor in the air. Mixing metal hydrides with water, as shown above, allows for the metal to be constantly bonded, increasing stability.
Environmental Benefit
Hydrogen is now a factor in the clean and renewable energy debate. Hydrogen gas can be burned in internal combustion engines or run through a fuel cell to produce electricity with the only byproduct being water. Hydrogen is an energy carrier and not a primary source of energy. Hydrogen would be useful in nearly anything that requires a portable energy source. This includes cars, trucks, airplanes, boats, or any other type of mechanical transportation as well as items such as portable electronics. If the hydrogen were produced using renewable technologies such as wind or solar energy then the total amount of greenhouse gases emitted would drop dramatically. The main goal of using hydrogen as an energy carrier is to reduce total greenhouse gas emissions across the globe.
Hydrogen Safety
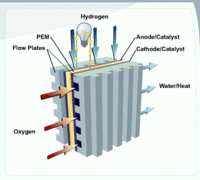
All fuels have some level of safety risk. Many consider hydrogen to be an unsafe explosive gas and reference the burning Hindenburg as an example. In fact, it was not the hydrogen of the Hindenburg that initially caught fire but the highly combustible fabric of the airship. Hydrogen is not toxic to humans, and if released, will spread rapidly into the atmosphere due to its low density, reducing risk of asphyxiation and explosion. In fact, hydrogen is less flammable than gasoline and requires a higher temperature for autoignition. Other safety issues are specific to hydrogen because of its particular properties and storage mediums. Hydrogen burns with a clear, nearly invisible flame, requiring special flame detectors. When stored under high pressures or at very low temperatures, additional precautions are necessary for its safe handling.
When considering metal hydrides, consideration of the toxicity of both the hydride itself and the resulting byproduct, flammability, as well as the temperature and pressure required for storage are necessary for safe handling. Most metal hydrides are non-toxic to humans but many of the resulting metal hydroxide compounds can be very acidic, corrosive, and generally dangerous, though this varies widely based upon the specific metal hydrides used. Some hydrides are flammable near room temperature, making them dangerous to handle. From a safety perspective, the best metal hydride and its related hydroxide byproduct would both be nontoxic, have a high ignition temperature, and could be stored at room temperature near atmospheric pressure.
Challenges to Metal Hydride Hydrogen Storage
There is a long road ahead when considering the amount of research and implementation that will be required before metal hydrides can be used as a viable alternative energy carrier.
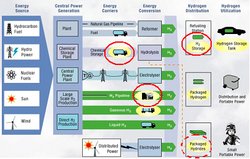
The Department of Energy desires that hydrogen fuelled vehicles perform similarly to gasoline fueled vehicles and has therefore set the following standards for hydrogen fuelled vehicles, all of which will be difficult for metal hydrides to meet:
- gravimetric energy density: 2 kWh/kg
- volumetric energy density: 1.5 kWh/l
- H2 storage capacity (mass fraction) of 6 wt% (on a system basis)
- operating temperature: -30 to +50oC
- re-fueling time < 5 minutes
- re-fueling rate: 1.5 kg H2/min
- recoverable amount of hydrogen: 90%
Low Hydrogen Capacity
Metal hydrides have a relatively low hydrogen capacity, meaning that a large amount of the hydride chemical would be necessary to extract a useful amount of hydrogen gas.
Temperature and Pressure
Metal hydrides will react with water to provide hydrogen gas but will do so only under specific temperature and pressure conditions. It is often necessary to increase the pressure and temperature (inputting additional energy) in order to allow for the reaction to begin. Most metal hydride reactions are heat producing [exothermic] reactions and will be able to sustain themselves until the limiting reactant has been used up.
Infrastructure Issues
Like other types of hydrogen storage platforms, large infrastructure changes will be necessary before metal hydrides can be used. For example, if these compounds were used as vehicular fuel, engines and fueling systems would have to be designed much differently. Also, all fueling stations would need to be overhauled for safe distribution.
Cost
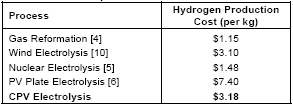
Cost is a limiting factor in much of the discussion of hydrogen as an alternative energy source. A gallon of gasoline contains approximately the same amount of energy as a kilogram of hydrogen. Gasoline currently costs over three dollars per gallon in the United States yet hydrogen fuel is still not economically competitive. Metal hydrides are one of the more expensive forms of hydrogen storage but costs are likely to come down with additional research and increased scale. A cost comparison of the some different energy sources for hydrogen is shown below. The Department of Energy set a hydrogen cost goal of $2.00-3.00/gge (gallons of gasoline equivalent, delivered, untaxed, 2005$, by 2015) in 2005.
Reversibility
The best way to reduce the cost and limit the environmental impact of metal hydride hydrogen storage is to prove reversibility of the reactions. As shown above, metal hydride reactions with water result in metal hydroxide byproducts. If researchers are able to determine a way to recycle the hydroxide byproduct back into the hydride reactant, both costs and environmental impacts will be reduced as the same metal atoms will be able to be used over and over again. Current research has shown that these reactions appear to be possible but they have yet to be proven over hundreds or thousands of cycles.
Current/Future Uses and Niche Markets for Metal Hydrides
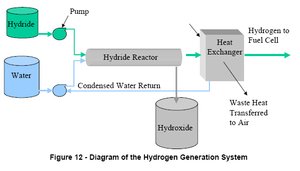
Research is continuing to find more applications for metal hydrides including nearly every facet of our lives that require a mobile energy source such as vehicles, electronics, and boats. Rechargeable batteries have already been developed using nickel metal hydride. Multiple companies have developed proprietary metal hydride canisters that can provide portable fuel for nearly any application. The debate is still ongoing as to which hydrides are best and how to implement their use
Use as a Vehicular Fuel
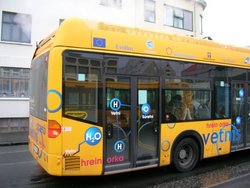
Multiple buses in Reykjavik, Iceland now run exclusively on hydrogen. Other projects are underway in hopes of converting the fishing fleets in Iceland to hydrogen power as well. The current uses of hydrogen as a vehicular fuel parallels the belief that to become a viable method of energy storage, it will be necessary to first use the fuel in niche markets that use a fleet of vehicles such as tucking companies or the postal service. This idea will reduce the cost of implementation by requiring that only one central fueling station be built for the fleet. Though hydrogen can be burned in an internal combustion engine, additional engineering would be necessary for cars to be able to store the metal hydride and release the hydrogen gas.
Some Specific Metal Hydrides
Hydrides can form with multiple alkaline and transition metals. Each of the hydrides listed below can undergo a similar reaction with water to produce hydrogen and a hydroxide byproduct. It is also possible to remove the hydrogen from the solid hydride through the application of heat. The gravimetric density (weight percent of hydrogen to the total weight of the system) is provided for each of the metal hydrides surveyed below. This is a necessary consideration when considering the use of metal hydrides in things like vehicles where space is limited. The pros and cons of the specific metal hydrides are also considered.
Lithium Hydride
Lithium hydride will react at room temperature and is therefore difficult to handle. When reacted with water, it forms the lithium hydroxide byproduct which is very corrosive. Gravimetric density = 25.2%. The hydrogen producing reaction is as follows: LiH + H2O → LiOH + H2.
Magnesium Hydride
Magnesium hydride requires increased temperatures and/or atmospheric pressure in order to react with water to release hydrogen gas. The resulting byproduct, magnesium hydroxide, is benign and commonly known as milk of magnesia. Gravimetric Density = 15.2%. The hydrogen producing reaction is as follows: MgH2 + 2H2O → Mg(OH)2 + 2H2.
Calcium Hydride
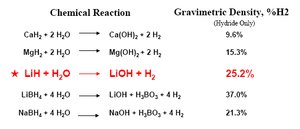
Calcium hydride and is incredibly flammable and will react with water at room temperature creating safety hazards for handling. The resulting calcium hydroxide byproduct is also known as slaked lime and is a known skin irritant. Gravimetric Density = 9.6%. The hydrogen producing reaction is as follows: CaH2 + 2H2O → Ca(OH)2 + 2H2.
More Complex Metal Hydrides
Such as LiMg(AlH4)3 and Na3AlH6 are also being considered by researchers. These compounds appear to be able to increase the gravimetric density of hydrogen but the reversibility of these reactions is much more difficult.
References
- Smith, R. L.; Miser, J. W. (1963). Compilation of the properties of lithium hydride. NASA.
- Empirical laws for hybrid combustion of lithium hydride with flourine in small rocket engines. Ntrs.nasa.gov.
- Gawley, R. E.; Davis, A. "Calcium Hydride" in Encyclopedia of Reagents for Organic Synthesis (Ed: L. Paquette) 2004, J. Wiley & Sons, New York. doi:10.1002/047084289X.rc005.
- Adlam G.H.J. and Price L.S., A Higher School Certificate Inorganic Chemistry, John Murray, London, 1940