Windscale Fire
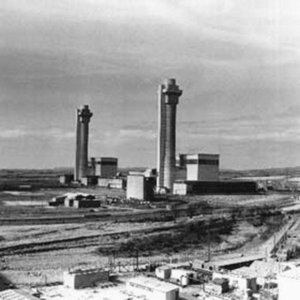
Topics: |
On October 10, 1957, the core of the Windscale nuclear reactor (which was being used for research and defense purposes) in Cumbria, England caught fire and burned for nearly two days before being extinguished. The incident resulted in the release of radioactive material into the surrounding area that spread across much of northern Europe and western Scandinavia. Within days of the accident, the British government initiated an official inquiry into the causes of the fire. Although no serious environmental or health effects were noted in the aftermath of the event, it was nonetheless the largest accidental release of radioactive material in the history of the British nuclear industry. Prior to information becoming available regarding the Soviet nuclear program, the Windscale fire was considered the worst nuclear disaster before Three Mile Island in 1979 and Chernobyl in 1986.
Contents
Brief background of the British nuclear weapons program
At the onset of World War II in Europe, American scientists began to organize a project aimed at exploiting the newly discovered nuclear fission process for military purposes, as it was known that German scientists were also working on a similar enterprise. By 1943 the Manhattan Project had become a combined policy committee consisting of the United States, the United Kingdom, and Canada. The objective of this cooperative effort, which called for a joint technology-exchange program, was to develop a nuclear weapon (an atomic bomb) before the Germans.
After the war, the United States government passed legislation that closed its nuclear weapons program to all other countries, despite the participation of many British scientists in the Manhattan Project. As a result, the United Kingdom’s ability to keep pace in the emerging arms race of the post-World War II decade was severely jeopardized. Not wanting to defer its status as a world power in favor of the United States and the Soviet Union, Britain hastened to develop its own nuclear weapons program as quickly as possible
The Windscale Piles
The British government chose the village of Seascale in Cumbria, England as the site for the two Windscale nuclear reactors. Built relatively quickly from 1947 to 1951, the reactors – known as Windscale Pile 1 and Windscale Pile 2 – were housed separately in large, concrete buildings only a few hundred feet apart. Following the decision in January 1947 for Britain to build its own nuclear weapons, the Windscale Piles’ main function was to produce the plutonium necessary for said weapons.
Reactor core design
Each Pile at Windscale housed a 2,000-ton solid graphite core and was fueled by 180 tons of uranium (Uranium enrichment) metal fabricated into over 70,000 aluminum-encased rods. These rods were individually fed into one of 3,440 horizontal channels located in the front of the core and were pushed all the way through the core in order to undergo neutron irradiation and produce plutonium-239. The spent fuel was eventually dumped into a water duct for cooling prior to processing and Pu-239 extraction. As this plutonium was intended for weapons purposes, the consumption of the uranium fuel was kept as low as possible in order to reduce production of the heavier plutonium isotopes (Pu-240 or Pu-241, for instance).
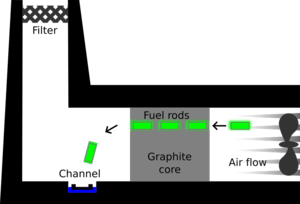
Additionally, the reactors were used to manufacture polonium-210 and tritium (accomplished by irradiating bismuth oxide and a magnesium-lithium alloy, respectively); these were used as fission triggers in nuclear weapons. The reactors also produced radioisotopes, such as cobalt-60, for industrial and medical use. Cartridges containing these target materials were placed in isotope channels that ran through the graphite core parallel to the fuel channels and subjected to neutron irradiation.
Wigner Energy
Shortly before the reactors were built, Hungarian physicist Eugene Wigner discovered that when graphite is bombarded by high-energy neutrons, carbon nuclei are displaced in the crystalline lattice because the kinetic energy of the neutrons is greater than the binding energy in the atoms' electrons. Displaced, or interstitial, atoms eventually come to rest in non-ideal locations – that is, not along the symmetrical lines of the lattice. If enough interstitial atoms are allowed to accumulate within the graphite, the resulting buildup of energy (subsequently termed Wigner Energy) poses a risk of escaping in a sudden rush of heat. Since the reactor cores were made of solid graphite and underwent neutron irradiation on a regular basis, it was necessary to find a means of safely releasing the energy stored inside it.
The solution was a process of controlled heating, called an annealing cycle, during which time the core was heated beyond its normal operating temperature to 250-270 degrees Celsius. As the temperature of the graphite rose, the atoms could slip back into place and the stored energy could be released gradually as heat. Cooling fans then blew air through the reactor and pushed the hot air out of the back of the core and up the 400-foot tall chimneys. Since radioactive material was present in the exhausted air, filters were added at the tops of the stacks.
The accident
Ignition
On October 7, 1957, operators initiated an annealing (controlled heating) cycle for Windscale Pile 1 by reducing airflow into the core. The next day, they slowly increased the power to the reactor in order to raise the temperature of the core, but it soon became apparent that a gradual release of energy from the graphite was not occurring as it should. In response, the operators further increased power in the hopes of accelerating the annealing process, but because the thermocouples (thermometers) were not located in the hottest parts of the core, they were unaware that some areas were considerably hotter than others. All that was visible on the instruments was a moderate increase in temperature, which was to be expected during annealing.
By October 9, the highest recorded temperature in the core had exceeded the permitted maximum of 400 degrees Celsius. Though the temperature was supposed to fall as the energy release ended, fan dampers were nonetheless opened for 15 minutes to induce a cooling flow of air through the reactor. This succeeded in removing much of the excess heat, but one thermocouple indicated that the temperature continued to rise in the core.
Early in the morning of October 10, operators noticed that the temperature had not dropped at all from the previous day. It was then that workers in the control room realized that the radiation monitoring devices that measured activity at the top of the discharge stack were at full-scale reading; the extremely overheated graphite core and uranium fuel rods had been burning in the air coolant, and their byproducts were pushed up and out of the stack.
The fire
Unable to examine the pile with a remote scanner, operators had to don protective gear and physically go to the front face (the charge face) of the reactor to retrieve an inspection plug. It was then that they discovered that the uranium cartridges in four fuel channels were “glowing bright cherry red.” There was now no doubt that the core was dangerously overheated and the fuel elements were on fire and had been for almost 48 hours. Soon more red-hot fuel cartridges were found glowing in the channels at the rear of the reactor, and flames were visibly licking at the reinforced concrete containment – concrete whose specifications required that it be kept below a certain temperature to prevent disintegration and collapse. Further exacerbating the situation was the operators’ inability to discharge the overheated and burning uranium rods: the metal aluminum canisters that housed them had been distorted by thermal expansion and could not be removed from the channels.
Firefighting attempts
Operators first tried to extinguish the blaze by turning the cooling fans to full power and blasting air into the reactor core, but this only fanned the flames. The decision was then made to use scaffolding poles to ram the damaged fuel cartridges through the reactor and into the water duct behind it. No matter how much force was applied, however, the rods refused to budge, and the poles were withdrawn with their ends glowing red-hot. At one point, a pole was returned dripping with molten irradiated uranium.
The next step was to try to quench the fire by injecting liquid carbon dioxide into the reactor. Twenty-five tons of liquid CO2 was rigged up to the charge face of Pile 1, but the fire inside the reactor was so hot that it stripped the oxygen from whatever carbon dioxide could be applied.
By the next day, eleven tons of uranium were ablaze. Temperatures inside the reactor core were extreme: one thermocouple registered 1,300 degrees Celsius, which was over three times the prescribed maximum. Additionally, the concrete biological containment surrounding the reactor was in severe danger of collapsing because of the heat. Faced with this potentially deadly crisis, operators finally decided to flood the core with water. This solution, itself a dangerous risk to take, was considered a last resort for good reason: molten metal oxidizes when it comes into contact with water. Stripping the oxygen from water molecules leaves free hydrogen, which could mix with the incoming air and explode, tearing open the already weakened containment.
A dozen nozzle-less hoses were hauled to the charge face of the reactor and fed into the fuel channels about a meter above the heart of the fire. All cooling and ventilating air entering the reactor was shut off in order to minimize the effect of a possible explosion. The water was turned on and as the pressure gradually increased, the flames leaping from the discharge face slowly died away. Operators continued to pump water into the core for the next 24 hours until the reactor was completely cold.
The aftermath
The limited instrumentation of the reactor at Windscale Works provided little information regarding the quantity of radioactive material released. Subsequently, discharge measurements were based on environmental evidence taken from surrounding areas, ranging from northwestern England to mainland Europe.
Local environmental and health effects
Outdoor air samples were routinely collected following the accident, and continuous radiation measurements were recorded at four locations along the perimeter fence. These monitors responded to gamma radiation from airborne and deposited radionuclides and showed peaks in the emission rate occurring during the evening of October 10 and the early morning of October 11. Ground deposition was dominated by iodine-131, but soil cores also showed sparse determinations of cesium-137.
Specific studies to identify ecological change were not undertaken. As the deposition from the Windscale accident was dominated by 131I, which has a half-life of only 8 days, the fallout’s long-term effect on environmental radionuclide inventories in the vicinity has been limited to a discernible deposition of 137Cs on the high ground about 20 kilometers from the site.
At the time of the accident, there was not yet an established protocol in place governing the limitation of radiation dose to the public; existing International Commission on Radiological Protection (ICRP) recommendations dealt only with continuous intake by reactor workers. In addition, the particulate filters at the top of the stacks somewhat limited radioactive release from the reactor. Therefore, evacuation from the adjacent village of Seascale was not deemed necessary after the fallout.
In the days following the accident, samples were taken from surrounding soil, herbage, food, and water. Monitoring indicated trace amounts of iodine-131, which causes thyroid cancer, and cesium-137 in dried milk. Milk distribution within 500 km2 was subsequently banned for a month, the milk diluted thousand-fold and dumped into the Irish Sea. Restrictions were lifted progressively as measurements showed that concentrations of 131I and 137Cs were no longer of concern.
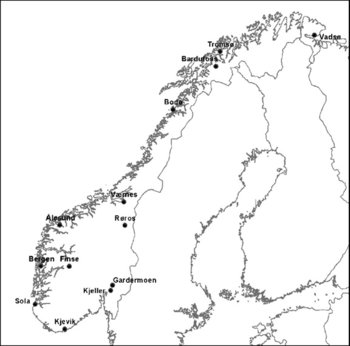
International environmental effects
From Cumbria, the radioactive plume from Windscale traveled in a southeasterly direction. Given the extensive reporting of the accident in the media, increased radioactive activity was detected at many sites in mainland Europe and in Scandinavia.
Due to its location, Norway was the most prone to receiving considerable levels of radioactive fallout during the nuclear era. The Norwegian Defense Research Establishment (FFI), using a network of filtration-based air and precipitation monitoring systems, conducted the monitoring of these fallouts.
On October 15-16, nuclear monitoring stations at Bergen and Sola registered increased levels of beta radiation in the vicinity; this was also the case a day later at Værnes. East of Bergen at Finse, no obvious elevation of radioactivity was recorded. Rainwater samples taken at Kjevik exhibited an increase in beta activity between October 10-20, and high rainfall during this time contributed to an elevation in deposited activity. However, rainwater at Bardufoss, in northern Norway, did not show an appreciable increase in radioactivity.
The presence of radioactivity on the southern and western coasts of Norway but not inland is largely explained by the Hardangervidda Mountains, which separate the coastal and inland stations. The stations to the west of these mountains experience the highest precipitation in Norway, and as a result the winds, which blow from the west year-round, deposit most of their radioactivity in areas of high precipitation on the western coast. Consequently, air masses moving over the inland stations have lower radioactivity.
Reaction
The accident at Windscale Pile 1 was widely reported in the media. Most reports labeled the incident a “mishap,” and many reassured readers that radioactive emission levels were “negligible” and “not dangerous.” Nonetheless, the Windscale fire had wide-reaching consequences with regards to the organization of the nuclear industry in the United Kingdom, with particular emphasis on the implementation of safety measures. In the aftermath, the Authority Health and Safety Board established itself as a separate unit functioning within the United Kingdom Atomic Energy Authority. This ultimately led to the formations in 1970 of the National Radiological Protection Board, which publicly disseminated information about protection from radiological hazards, and the Nuclear Installations Inspectorate, which encouraged and enforced safe working environments. Thus, it was enormous public response that most contributed to the development of radiation protection criteria in nuclear plants.
Board of Inquiry
Under the chairmanship of celebrated scientist William Penney, the government tasked an official Board of Inquiry to investigate the causes of the Windscale fire. From October 17-25, 1957, the Board convened and created the “Penney Report,” which provided four conclusions:
- The primary cause of the accident was the second nuclear heating during the annealing process of October 8, applied too soon and too rapidly.
- Steps taken to deal with the accident were "prompt and efficient and displayed considerable devotion to duty on the part of all concerned."
- Measures taken to deal with the consequences of the accident were adequate. There was "no immediate damage to the health of any of the public or of the workers."
- A more detailed and technical assessment of the incident was needed, meaning organizational changes, clearer responsibilities for health and safety, and a better definition of radiation dose limits were necessary.
The original report was submitted to Parliament in November 1957 and kept “absolutely confidential” for thirty years, until being released to the Public Record Office in January 1988. At that time, major discrepancies emerged between the original version and the edition that the public had heretofore been privy to. In the former, Penney had named as the primary cause of the accident not the abovementioned first conclusion, but “misjudgments by the Windscale staff, poor organization, and faulty safety instruments.” Prime Minister Harold Macmillan justified the cover-up by arguing that adverse publicity would have threatened the development of British nuclear weapons capabilities and its atomic energy program, both of which he deemed vital in light of the concurrent cold war arms race. Full disclosure of the contents of the report would have provided valuable material to American interests opposed to United States legislation permitting collaboration with Britain on military applications of atomic energy.
Salvage operations
Windscale Pile 1 was completely unsalvageable. Where possible, the uranium fuel rods were removed from the graphite core, which was still slightly warm as a result of continuing nuclear reactions, and the reactor’s concrete bioshield was sealed and left intact. Over 8,000 fire-damaged fuel cartridges and isotope canisters remain in the pile. Windscale Pile 2, though undamaged by the fire that tore through its counterpart, was considered too unsafe for further use and shut down shortly after the accident.
No air-cooled nuclear reactors have been built since the Windscale Piles. A cleanup of the site, which includes the final removal of fuel from the damaged reactor, is scheduled to begin in 2008 and is expected to cost upwards of $30 million.
References
"Atom Scare Halts Milk." New York Times. 13 October 1957. ProQuest Historical Newspapers. ProQuest. Boston University Lib. System, Boston, MA. 8 Oct 2008 <http://www.proquest.com/ezproxy.bu.edu>.
Bergan, T., M. Dowdall, and Ø.G. Selnæs. "On the occurrence of radioactive fallout over Norway as a result of the Windscale accident, October 1957." Journal of Environmental Radioactivity 99 (2008): 50-61. ScienceDirect. Boston University Lib. System, Boston, MA. 4 Oct 2008 <http://www.sciencedirect.com>.
Dwyer, Paul. "Windscale: A nuclear disaster." BBC News. 5 Oct 2007 <http://news.bbc.co.uk/2/hi/sciences/natures/7030281.stm>. 30 Sept 2008.
Garland, J.A. and R. Wakeford. "Atmospheric emissions from the Windscale accident of October 1957." Atmospheric Environment 41 (2007): 3904-3920. ScienceDirect. Boston University Lib. System, Boston, MA. 4 Oct 2008 <http://www.sciencedirect.com>.
Jones, Steve. "Windscale and Kyshtym: a double anniversary." Journal of Environmental Radioactivity 99 (2008): 1-6. ScienceDirect. Boston University Lib. System, Boston, MA. 4 Oct 2008 <http://www.sciencedirect.com>.
Lohrs, Steve. "Britian Suppressed Details of '57 Atomic Disaster." New York Times. 2 Jan 1988: 3. ProQuest Historical Newspapers. ProQuest. Boston University Lib. System, Boston, MA. 6 Oct 2008 <http://www.proquest.com/ezproxy.bu.edu>.
Nelson, N., K.P. Kitchen, and R.H. Maryon. "A study of the movement of radioactive material discharged during the Windscale fire in October 1957." Atmospheric Environment 40 (2005): 58-75. ScienceDirect. Boston University Lib. System, Boston, MA. 3 Nov 2008 <http://www.sciencedirect.com>.
"Red-Hot Uranium Worries Britons." New York Times. 12 Oct 1957: 2. ProQuest Historical Newspapers. ProQuest. Boston University Lib. System, Boston, MA. 8 Oct 2008 <http://www.proquest.com/ezproxy.bu.edu>.
Wakeford, Richard. "The Windscale reactor accident -- 50 years on." Journal of Radiological Protection 27 (2007): 211-215. IOP Electronic Journals. 8 Dec 2008 <http://www.iop.org/EJ/abstract/0952-4746/27/3/E02/>.
"Windscale fire." Wikipedia, The Free Encyclopedia. 31 Oct 2008, 21:49 UTC. 8 Dec 2008 <http://en.wikipedia.org/w/index.php?title=Windscale_fire&oldid=248894318>.