Absorption of toxicants
Introduction
Absorption is the process whereby toxicants gain entrance to the body. Ingested and inhaled materials, nonetheless, are considered outside the body until they cross the cellular barriers of the gastrointestinal tract or the respiratory system. To exert an effect on internal organs a toxicant must be absorbed, although such local toxicity as irritation, may occur.
Absorption varies greatly with specific chemicals and with the route of exposure. For skin, oral or respiratory exposure, the exposure dose (or, "outside" dose) is usually only a fraction of the absorbed dose (that is, the internal dose). For substances injected or implanted directly into the body, exposure dose is the same as the absorbed or internal dose.
Several factors affect the likelihood that a foreign chemical or, xenobiotic, will be absorbed. The most important are:
- route of exposure;
- concentration of the substance at the site of contact; and
- chemical and physical properties of the substance.
The relative roles of concentration and properties of the substance vary with the route of exposure. In some cases, a high percentage of a substance may not be absorbed from one route whereas a low amount may be absorbed via another route. For example, very little DDT powder will penetrate the skin whereas a high percentage will be absorbed when it is swallowed. Due to such route-specific differences in absorption, xenobiotics are often ranked for hazard in accordance with the route of exposure. A substance may be categorized as relatively non-toxic by one route and highly toxic via another route.
The primary routes of exposure by which xenobiotics can gain entry into the body are:
Other routes of exposure—used primarily for specific medical purposes—are:
For a xenobiotic to enter the body (as well as move within, and leave the body) it must pass across cell membranes (cell walls). Cell membranes are formidable barriers and major body defenses that prevent foreign invaders or substances from gaining entry into body tissues. Normally, cells in solid tissues (for example, skin or mucous membranes of the lung or intestine) are so tightly compacted that substances can not pass between them. Entry, therefore, requires that the xenobiotic have some capability to penetrate cell membranes. Also, the substance must cross several membranes in order to go from one area of the body to another.
In essence, for a substance to move through one cell requires that it first move across the cell membrane into the cell, pass across the cell, and then cross the cell membrane again in order to leave the cell. This is true whether the cells are in the skin, the lining of a blood vessel, or an internal organ (for example, the liver). In many cases, in order for a substance to reach its site of toxic action, it must pass through several membrane barriers.
A foreign chemical will pass through several membranes before it comes into contact with, and can damage, the nucleus of a liver cell. Cell membranes (often referred to as plasma membranes) surround all body cells and are basically similar in structure. They consist of two layers of phospholipid molecules arranged like a sandwich (referred to as a "phospholipid bilayer"). Each phospholipid molecule consists of a phosphate head and a lipid tail. The phosphate head is polar/ That is, it is hydrophilic (attracted to water). In contrast, the lipid tail is lipophilic (attracted to lipid-soluble substances).
The two phospholipid layers are oriented on opposing sides of the membrane so that they are approximate mirror images of each other. The polar heads face outward and the lipid tails inward in the membrane sandwich, as illustrated in Figure 2.
The cell membrane is tightly packed with these phospholipid molecules—interspersed with various proteins and cholesterol molecules. Some proteins span across the entire membrane providing for the formation of aqueous channels or pores.
Some toxicants move across a membrane barrier with relative ease while others find it difficult or impossible. Those that can cross the membrane, do so by one of two general methods: either passive transfer or facilitated transport.
Passive transfer consists of simple diffusion (or osmotic filtration) and is "passive" in that there is no requirement for cellular energy or assistance.
Some toxicants can not simply diffuse across the membrane. They require assistance that is facilitated by specialized transport mechanisms. The primary types of specialized transport mechanisms are:
- facilitated diffusion;
- active transport; and
- endocytosis (phagocytosis and pinocytosis).
Passive transfer is the most common way that xenobiotics cross cell membranes. Two factors determine the rate of passive transfer:
- differences in concentrations of the substance on opposite sides of the membrane (substance moves from a region of high concentration to one having a lower concentration. Diffusion will continue until the concentration is equal on both sides of the membrane); and
- ability of the substance to move either through the small pores in the membrane or through the lipophilic interior of the membrane.
Properties of the chemical substance that affect its ability for passive transfer are:
- lipid solubility;
- molecular size; and
- degree of ionization (that is, the electrical charge of an atom).
Substances with high lipid solubility readily diffuse through the phospholipid membrane. Small water-soluble molecules can pass across a membrane through the aqueous pores, along with normal intracellular water flow.
Large water-soluble molecules usually can not make it through the small pores, although some may diffuse through the lipid portion of the membrane, but at a slow rate. In general, highly ionized chemicals have low lipid solubility and pass with difficulty through the lipid membrane.
Most aqueous pores are about 4 ångström (Å) in size and allow chemicals of molecular weight 100-200 to pass through. Exceptions are membranes of capillaries and kidney glomeruli that have relatively large pores (about 40Å) that allow molecules up to a molecular weight of about 50,000 (molecules slightly smaller than albumen which has a molecular weight of 60,000) to pass through.
Facilitated diffusion is similar to simple diffusion in that it does not require energy and follows a concentration gradient. The difference is that it is a carrier-mediated transport mechanism. The results are similar to passive transport but faster and capable of moving larger molecules that have difficulty diffusing through the membrane without a carrier. Examples are the transport of sugar and amino acids into red blood cells (RBCs), and into the central nervous system (CNS).
Some substances are unable to move with diffusion, unable to dissolve in the lipid layer, and are too large to pass through the aqueous channels. For some of these substances, active transport processes exist in which movement through the membrane may be against the concentration gradient: they move from low to higher concentrations. Cellular energy from adenosine triphosphate (ADP) is required in order to accomplish this. The transported substance can move from one side of the membrane to the other side by this energy process. Active transport is important in the transport of xenobiotics into the liver, kidney, and central nervous system and for maintenance of electrolyte and nutrient balance.
Many large molecules and particles can not enter cells via passive or active mechanisms. However, some may enter, as yet, by a process known as endocytosis.
In endocytosis, the cell surrounds the substance with a section of its cell wall. This engulfed substance and section of membrane then separates from the membrane and moves into the interior of the cell. The two main forms of endocytosis are phagocytosis and pinocytosis.
In phagocytosis (cell eating), large particles suspended in the extracellular fluid are engulfed and either transported into cells or are destroyed within the cell. This is a very important process for lung phagocytes and certain liver and spleen cells. Pinocytosis (cell drinking) is a similar process but involves the engulfing of liquids or very small particles that are in suspension within the extracellular fluid.
Contents
Gastrointestinal Tract
The gastrointestinal tract (GI tract, the major portion of the alimentary canal) can be viewed as a tube going through the body. Its contents are considered exterior to the body until absorbed. Salivary glands, the liver, and the pancreas are considered accessory glands of the GI tract as they have ducts entering the GI tract and secrete enzymes and other substances. For foreign substances to enter the body, they must pass through the gastrointestinal mucosa, crossing several membranes before entering the blood stream.
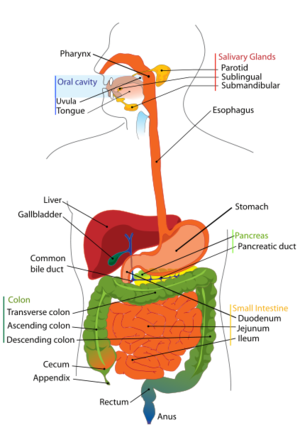
Substances must be absorbed from the gastrointestinal tract in order to exert a systemic toxic effect, although local gastrointestinal damage may occur. Absorption can occur at any place along the entire gastrointestinal tract. However, the degree of absorption is strongly site-dependent.
Three main factors affect absorption within the various sites of the gastrointestinal tract:
- type of cells at the specific site;
- period of time that the substance remains at the site; and
- pH of stomach or intestinal contents at the site.
Under normal conditions, xenobiotics are poorly absorbed within the mouth and esophagus, due mainly to the very short time that a substance resides within these portions of the gastrointestinal tract. There are some notable exceptions. For example, nicotine readily penetrates the mouth mucosa. Also, nitroglycerin is placed under the tongue (sublingual) for immediate absorption and treatment of heart conditions. The sublingual mucosa under the tongue and in some other areas of the mouth is thin and highly vascularized so that some substances will be rapidly absorbed.
The stomach, having high acidity (pH 1-3), is a significant site for absorption of weak organic acids, which exist in a diffusible, nonionized and lipid-soluble form. In contrast, weak bases will be highly ionized and therefore are absorbed poorly. Chemically, the acidic stomach may break down some substances. For this reason those substances must be administered in gelatin capsules or coated tablets, that can pass through the acidic stomach into the intestine before they dissolve and release their contents.
Another determinant that affects the amount of a substance that will be absorbed in the stomach is the presence of food. Food ingested at the same time as the xenobiotic may result in a considerable difference in absorption of the xenobiotic. For example, the LD50 for Dimethline (a respiratory stimulant) in rats is 30 mg/kg (or 30 parts per million) when ingested along with food, but only 12 mg/kg when it is administered to fasting rats.
The greatest absorption of chemicals, as with nutrients, takes place in the intestine, particularly in the small intestine. The intestine has a large surface area consisting of outward projections of the thin (one-cell thick) mucosa into the lumen of the intestine (the villi). This large surface area facilitates diffusion of substances across the cell membranes of the intestinal mucosa.
Since the intestinal pH is near neutral (pH 5-8), both weak bases and weak acids are nonionized and are usually readily absorbed by passive diffusion. Lipid soluble, small molecules effectively enter the body from the intestine by passive diffusion.
In addition to passive diffusion, facilitated and active transport mechanisms exist to move certain substances across the intestinal cells into the body, including such essential nutrients as glucose, amino acids and calcium. Also, strong acids, strong bases, large molecules, and metals (and some important toxins) are transported by these mechanisms. For example, lead, thallium, and paraquat (herbicide) are toxicants that are transported across the intestinal wall by active transport systems.
The high degree of absorption of ingested xenobiotics is also due to the slow movement of substances through the intestinal tract. This slow passage increases the length of time that a compound is available for absorption at the intestinal membrane barrier.
Intestinal microflora and gastrointestinal enzymes can affect the toxicity of ingested substances. Some ingested substances may be only poorly absorbed but they may be biotransformed within the gastrointestinal tract. In some cases, their biotransformed products may be absorbed and be more toxic than the ingested substance. An important example is the formation of carcinogenic nitrosamines from non-carcinogenic amines by intestinal flora.
Very little absorption takes place in the colon and rectum. As a general rule, if a xenobiotic has not been absorbed after passing through the stomach or small intestine, very little further absorption will occur. However, there are some exceptions, as some medicines may be administered as rectal suppositories with significant absorption. An example, is Anusol (hydrocortisone preparation) used for treatment of local inflammation which is partially absorbed (about 25%).
Respiratory Tract
Many environmental and occupational agents as well as some pharmaceuticals are inhaled and enter the respiratory tract. Absorption can occur at any place within the upper respiratory tract. However, the amount of a particular xenobiotic that can be absorbed at a specific location is highly dependent upon its physical form and solubility.
There are three basic regions to the respiratory tract:
- nasopharyngeal region;
- tracheobronchial region; and
- pulmonary region.
By far the most important site for absorption is the pulmonary region consisting of the very small airways (bronchioles) and the alveolar sacs of the lung.
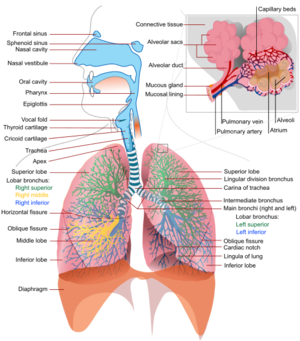
The alveolar region has a very large surface area (about 50 times that of the skin). In addition, the alveoli consist of only a single layer of cells with very thin membranes that separate the inhaled air from the blood stream. Oxygen, carbon dioxide and other gases pass readily through this membrane. In contrast to absorption via the gastrointestinal tract or through the skin, gases and particles, which are water-soluble (and thus blood soluble), will be absorbed more efficiently from the lung alveoli. Water-soluble gases and liquid aerosols can pass through the alveolar cell membrane by simple passive diffusion.
In addition to solubility, the ability to be absorbed is highly dependent on the physical form of the agent (that is, whether the agent is a gas/vapor or a particle). The physical form determines penetration into the deep lung.
A gas or vapor can be inhaled deep into the lung and if it has high solubility in the blood, it is almost completely absorbed in one respiration. Absorption through the alveolar membrane is by passive diffusion, following the concentration gradient. As the agent dissolves in the circulating blood, it is taken away so that the amount that is absorbed and enters the body may be quite large.
The only way to increase the amount absorbed is to increase the rate and depth of breathing. This is known as ventilation-limitation. For blood-soluble gases, equilibrium between the concentration of the agent in the inhaled air and that in the blood is difficult to achieve. Inhaled gases or vapors, which have poor solubility in the blood, have quite limited capacity for absorption. The reason for this is that the blood can become quickly saturated. Once saturated, blood will not be able to accept the gas and it will remain in the inhaled air and then exhaled.
The only way to increase absorption would be to increase the rate of blood supply to the lung. This is known as flow-limitation. Equilibrium between blood and the air is reached more quickly for relatively insoluble gases than for soluble gases.
The absorption of airborne particles is usually quite different from that of gases or vapors. The absorption of solid particles, regardless of solubility, is dependent upon particle size.
Large particles (>5 µM) are generally deposited in the nasopharyngeal region (head airways region) with little absorption. Particles 2-5 µM can penetrate into the tracheobronchial region. Very small particles (<1 µM) are able to penetrate deep into the alveolar sacs where they can deposit and be absorbed.
Minimal absorption takes place in the nasopharyngeal region due to the cell thickness of the mucosa and the rapid movement of gases and particles through the region. Within the tracheobronchial region, relatively soluble gases can quickly enter the blood stream. Most deposited particles are moved back up to the mouth where they are swallowed.
Absorption in the alveoli is quite efficient compared to other areas of the respiratory tract. Relatively soluble material (gases or particles) is quickly absorbed into systemic circulation. Pulmonary macrophages exist on the surface of the alveoli. They are not fixed and not a part of the alveolar wall. They can engulf particles just as they engulf and kill microorganisms. Some non-soluble particles are scavenged by these alveolar macrophages and cleared into the lymphatic system.
Some other particles may remain in the alveoli indefinitely. For example, coal dust and asbestos fibers may lead to black lung disease or asbestosis, respectively.
The nature of toxicity of inhaled materials depends on whether the material is absorbed or remains within the alveoli and small bronchioles. If the agent is absorbed and is also lipid soluble, it can rapidly distribute throughout the body passing through the cell membranes of various [[organ]s] or into fat depots. The time to reach equilibrium is even greater for the lipid soluble substances. Chloroform and ether are examples of lipid-soluble substances with high blood solubility.
Non-absorbed foreign material can also cause severe toxic reactions within the respiratory system. This may take the form of chronic bronchitis, alveolar breakdown (emphysema), fibrotic lung disease, and even lung cancer. In some cases, the toxic particles can kill the alveolar macrophages, which results in a lowering of the bodies' respiratory defense mechanism.
Dermal Route
In contrast to the thin membranes of the respiratory alveoli and the gastrointestinal villi, the skin is a complex, multilayer tissue. For this reason, it is relatively impermeable to most ions as well as aqueous solutions. It represents, therefore, a barrier to most xenobiotics. Some notable toxicants, however, can gain entry into the body following skin contamination.
For example, certain commonly used organophosphate pesticides have poisoned agricultural workers following dermal exposure. The neurological warfare agent, Sarin, readily passes through the skin and can produce quick death to exposed persons. Several industrial solvents can cause systemic toxicity by penetration through the skin. For example, carbon tetrachloride penetrates the skin and causes liver injury. Hexane can pass through the skin and cause nerve damage.
The skin consists of three main layers of cells:
- epidermis;
- dermis; and
- subcutaneous tissue.
The epidermis (and particularly the stratum corneum) is the only layer that is important in regulating penetration of a skin contaminant. It consists of an outer layer of cells, packed with keratin, known as the stratum corneum layer. The stratum corneum is devoid of blood vessels. The cell walls of the keratinized cells are apparently double in thickness due to the presence of the keratin, which is chemically resistant and an impenetrable material. The blood vessels are usually about 100 µM from the skin surface. To enter a blood vessel, an agent must pass through several layers of cells that are generally resistant to penetration by chemicals.
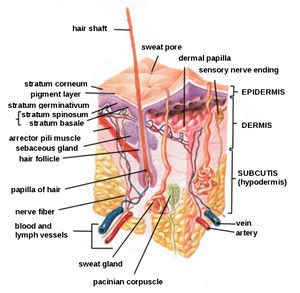
The thickness of the stratum corneum varies greatly with regions of the body. The stratum corneum of the palms and soles is very thick (400-600 µM) whereas that of the arms, back, legs, and abdomen is much thinner (8-15 µM). The stratum corneum of the axillary (underarm) and inquinal (groin) regions is the thinnest with the scrotum especially thin. As expected, the efficiency of penetration of toxicants is inversely related to the thickness of the epidermis.
Any process that removes or damages the stratum corneum can enhance penetration of a xenobiotic. Abrasion, scratching, or cuts to the skin will make it more penetrable. Some acids, alkalis, and corrosives can injure the stratum corneum and increase penetration to themselves or other agents. The most prevalent skin conditions that enhance dermal absorption are skin burns and dermatitis.
Toxicants move across the stratum corneum by passive diffusion. There are no known active transport mechanisms functioning within the epidermis. Polar and nonpolar toxicants diffuse through the stratum corneum by different mechanisms. Polar compounds (which are water-soluble) appear to diffuse through the outer surface of the hydrated keratinized layer. Nonpolar compounds (which are lipid-soluble) dissolve in and diffuse through the lipid material between the keratin filaments.
Water plays an important role in dermal absorption. Normally, the stratum corneum is partially hydrated (~7% by weight). Penetration of polar substances is about 10 times as effective as when the skin is completely dry. Additional hydration can increase penetration by 3-5 times which further increases the ability of a polar compound to penetrate the epidermis.
A solvent sometimes used to promote skin penetration of drugs is dimethyl sulfoxide (DMSO). It facilitates penetration of chemicals by an unknown mechanism. Removal of the lipid material creates holes in the epidermis. This results in a reversible change in protein structure due to substitution of water molecules.
Considerable species differences exist in skin penetration and can influence the selection of species used for safety testing. Penetration of chemicals through the skin of the monkey, pig, and guinea pig is often similar to that of humans. The skin of the rat and rabbit is generally more permeable whereas the skin of the cat is generally less permeable. For practical reasons and to assure adequate safety, the rat and rabbit are normally used for dermal toxicity safety tests.
In addition to the stratum corneum, small amounts of chemicals may be absorbed through the sweat glands, sebaceous glands, and hair follicles. Since these structures represent, however, only a very small percentage of the total surface area, they are not ordinarily important in dermal absorption.
Once a substance penetrates through the stratum corneum, it enters lower layers of the epidermis, the dermis, and subcutaneous tissue. These layers are far less resistant to further diffusion. They contain a porous, nonselective aqueous diffusion medium, that can be penetrated by simple diffusion. Most toxicants that have passed through the stratum corneum can now readily move on through the remainder of the skin and enter the circulatory system via the large numbers of venous and lymphatic capillaries in the dermis.
Other Routes of Exposure
In addition to the common routes of environmental, occupational, and medical exposure (oral, respiratory, and dermal), other routes of exposure may be used for medical purposes. Many pharmaceuticals are administered by parenteral routes. That is, by injection into the body usually via syringe and hollow needle.
Intradermal injections are made directly into the skin, just under the stratum corneum. Tissue reactions are minimal and absorption is usually slow. If the injection is beneath the skin, the route is referred to as a subcutaneous injection. Since the subcutaneous tissue is quite vascular, absorption into the systemic circulation is generally rapid. Tissue sensitivity is also high and thus irritating substances may induce pain and an inflammatory reaction.
Many pharmaceuticals, especially antibiotics and vaccines are administered directly into muscle tissue (the intramuscular route). It is an easy procedure and the muscle tissue is less likely to become inflamed compared to subcutaneous tissue. Absorption from muscle is about the same as from subcutaneous tissue.
Substances may be injected directly into large blood vessels when they are irritating or when an immediate action is desired, such as anesthesia. These are known as intravenous or intraarterial routes depending on whether the vessel is a vein or artery.
Parenteral injections may also be made directly into body cavities, rarely in humans but frequently in laboratory animal studies. Injection into the abdominal cavity is known as intraperitoneal injection. If it is injected directly into the chest cavity, it is referred to as an intrapleural injection. Since the pleura and peritoneum have minimal blood vessels, irritation is usually minimal and absorption is relatively slow.
Implantation is another route of exposure of increasing concern. A large number of pharmaceuticals and medical devices are now implanted in various areas of the body. Implants may be used to allow slow, time-release of a substance (e.g., hormones). In other cases, no absorption is desired. For example, for implanted medical devices and materials (e.g., artificial lens, tendons and joints, and cosmetic reconstruction).
Some materials enter the body via skin penetration as the result of accidents or violence (for example, weapons). The absorption in these cases is highly dependent on the nature of the substance. Metallic objects (such as bullets) may be poorly absorbed whereas more soluble materials that are thrust through the skin and into the body from accidents may be absorbed rapidly into the circulation.
Novel methods of introducing substances into specific areas of the body are often used in medicine. For example, conjunctival instillations (eye drops) are used for treatment of ocular conditions where high concentrations are needed on the outer surface of the eye, not possible by other routes.
Therapy for certain conditions require that a substance be deposited in body openings where high concentrations and slow release may be needed while keeping systemic absorption to a minimum. For these substances, the pharmaceutical agent is suspended in a poorly absorbed material such as beeswax with the material known as a suppository. The usual locations for use of suppositories are the rectum and vagina.
Reference
- National Library of MedicineToxicology Tutor II, Toxicogenetics, Adsorption
Disclaimer: This article is taken wholly from, or contains information that was originally published by, the National Library of Medicine. Topic editors and authors for the Encyclopedia of Earth may have edited its content or added new information. The use of information from the National Library of Medicine should not be construed as support for or endorsement by that organization for any new information added by EoE personnel, or for any editing of the original content. |